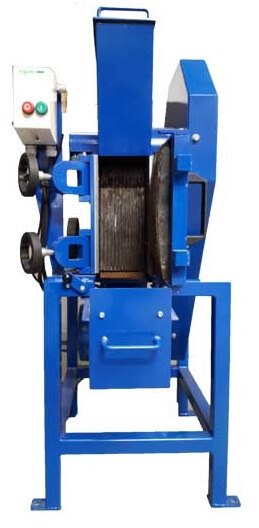
Hover
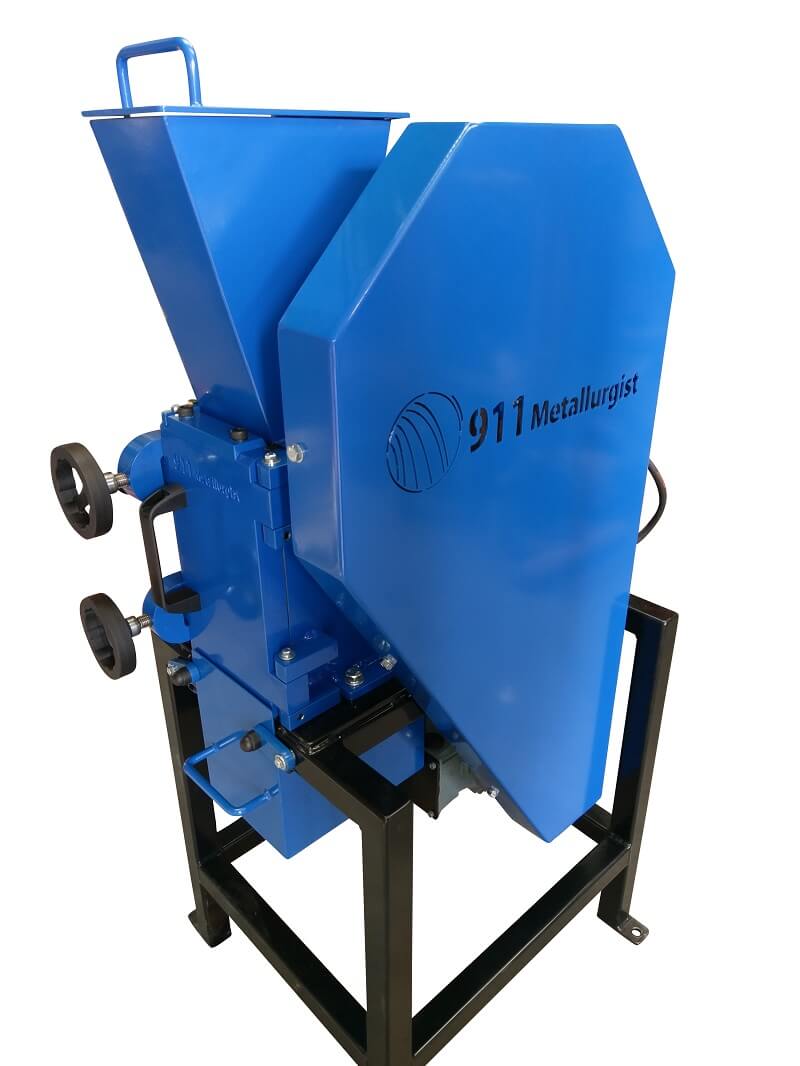
Hover
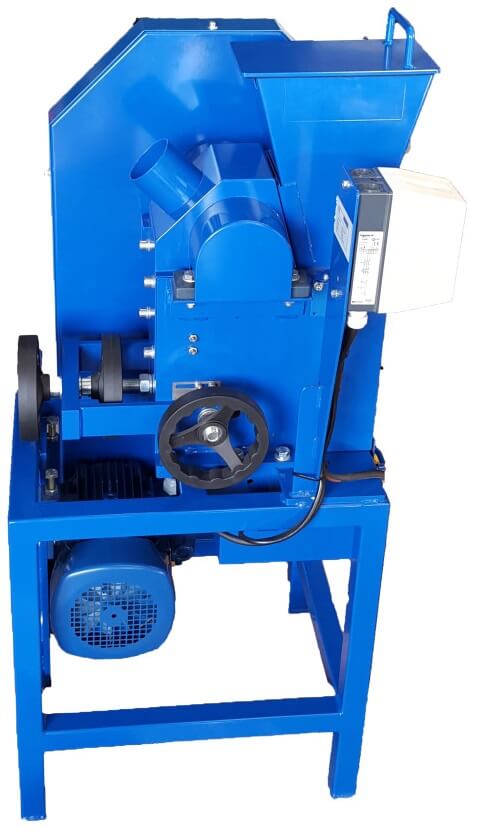
Hover
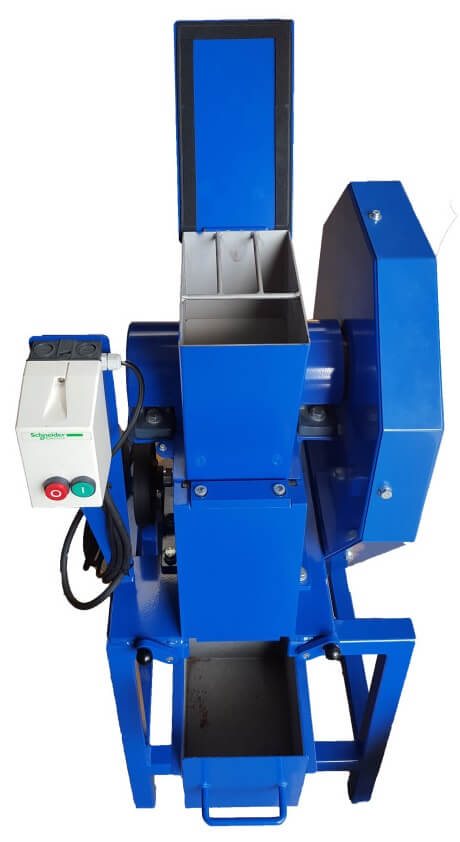
Hover
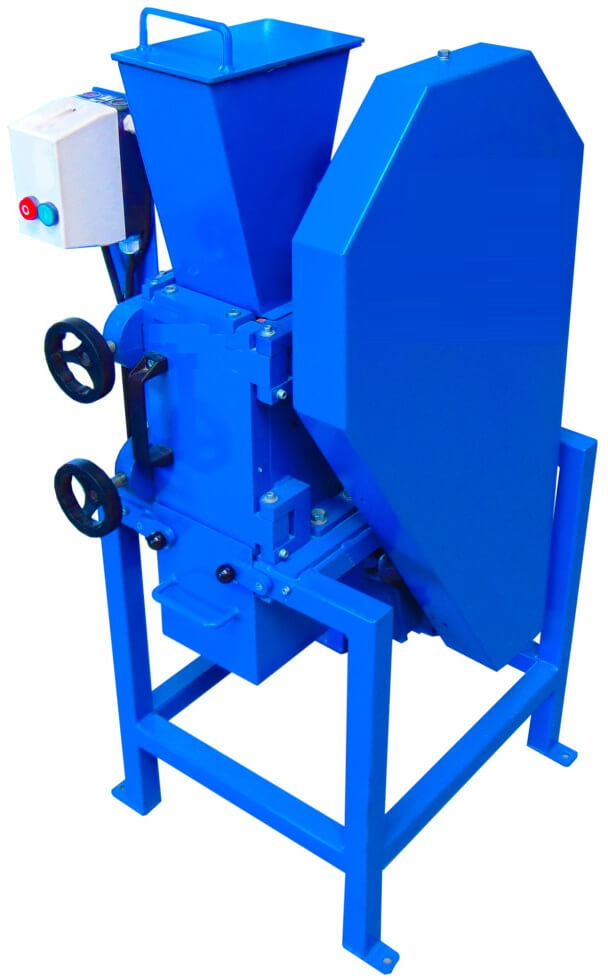
Hover
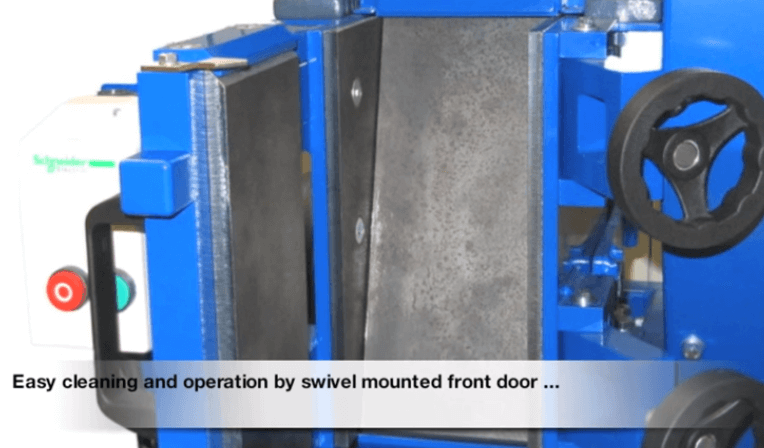
Hover
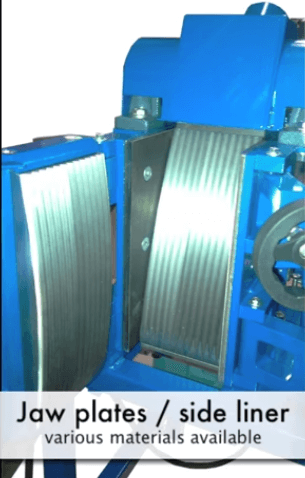
Hover
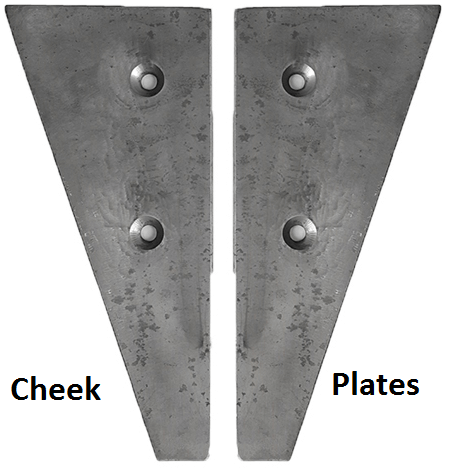
Hover
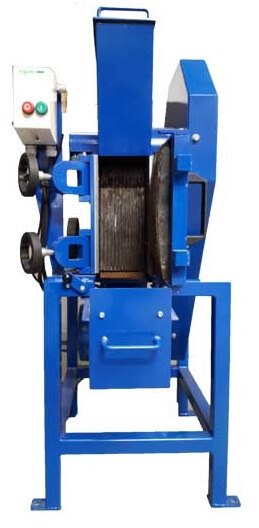
Hover
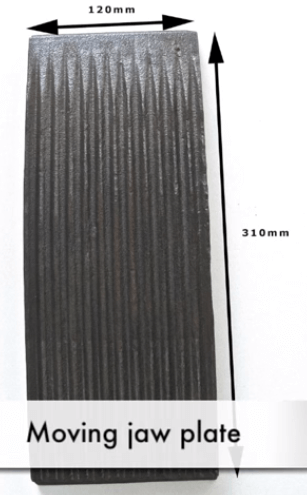
Hover
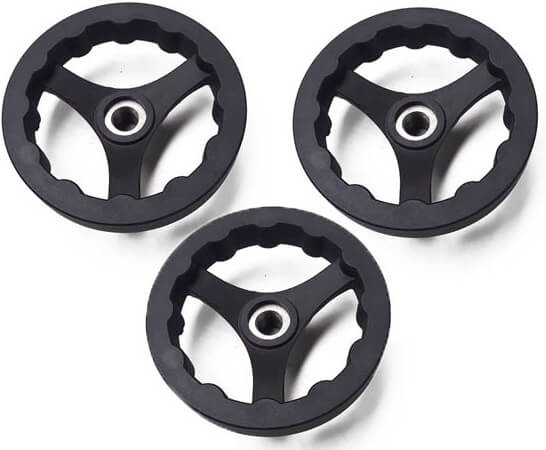
Hover
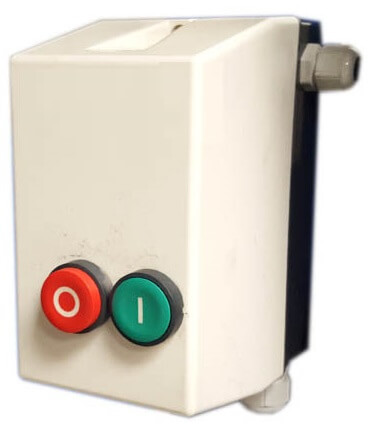
Hover
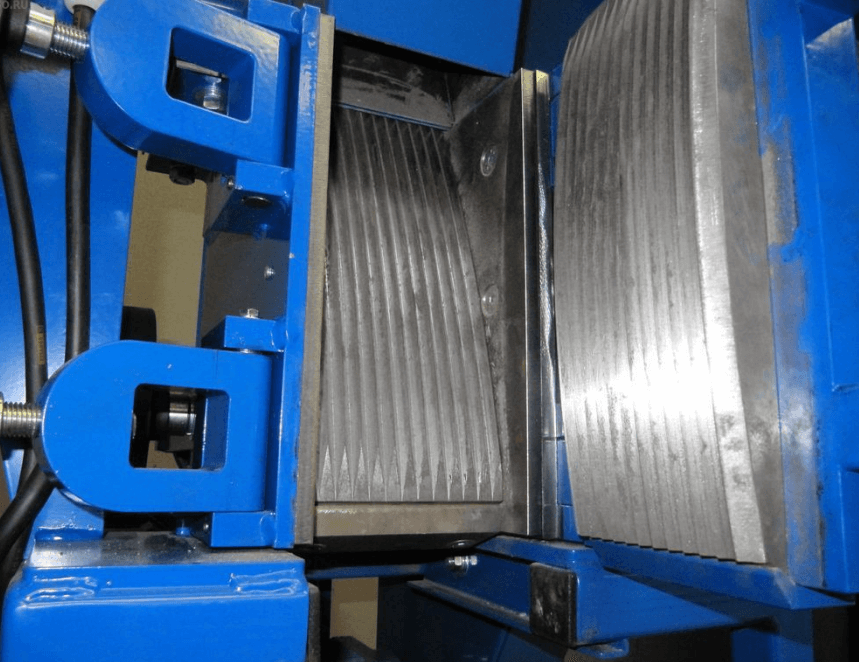
Hover
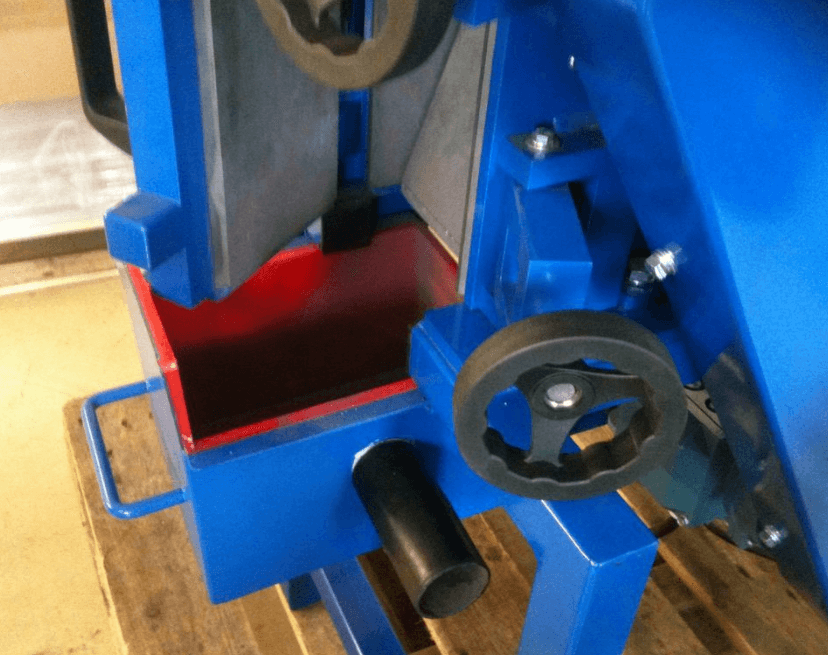
Hover
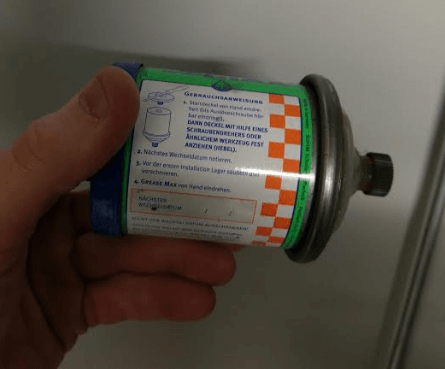
Hover
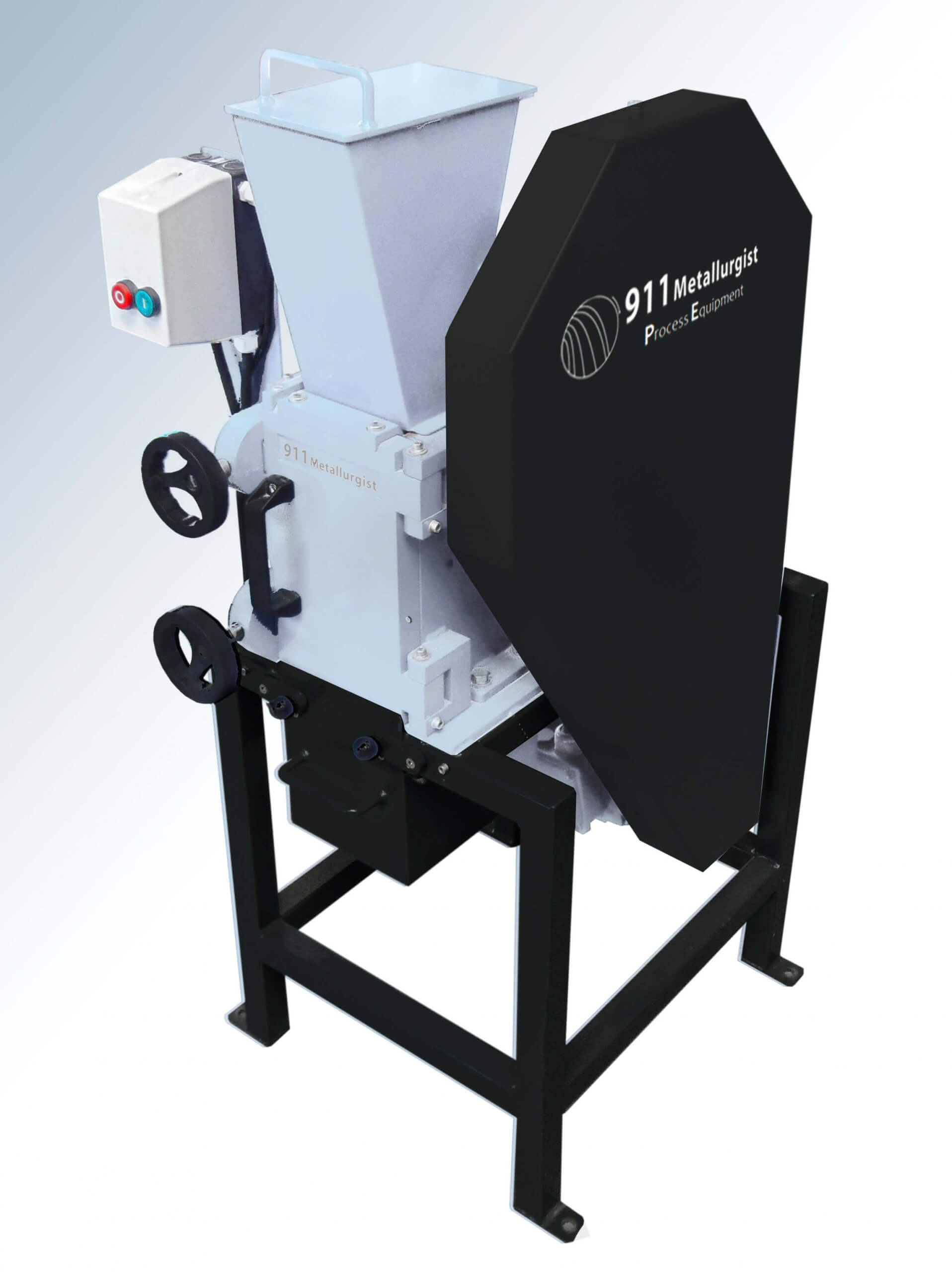
Hover

Hover

Hover
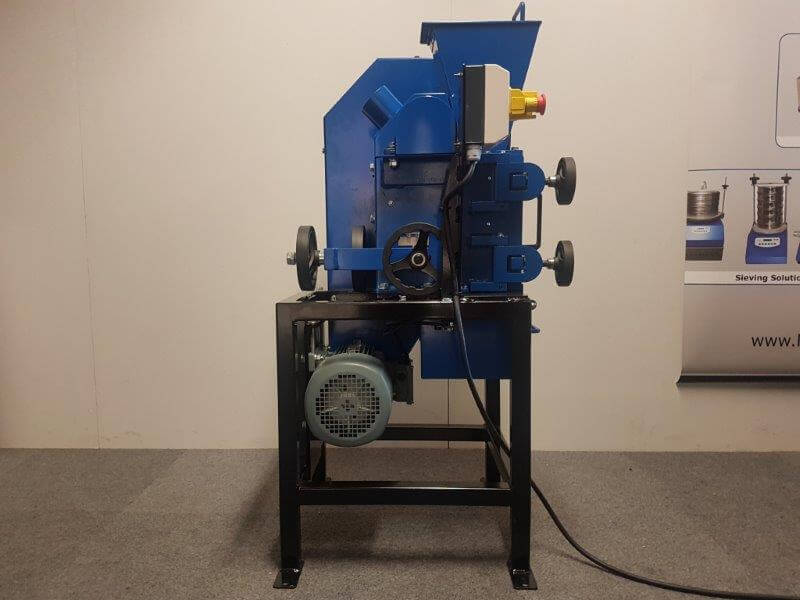
Hover
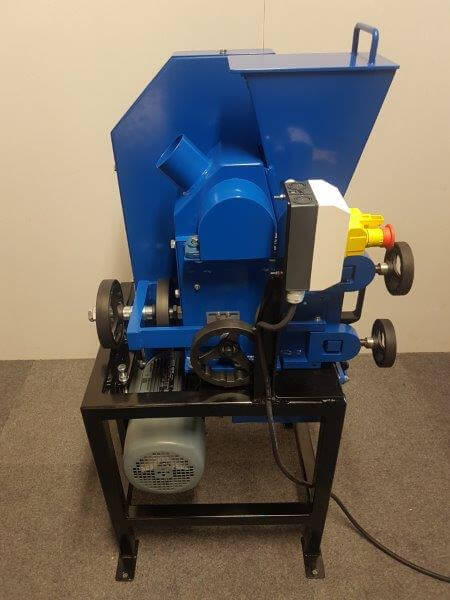
Hover
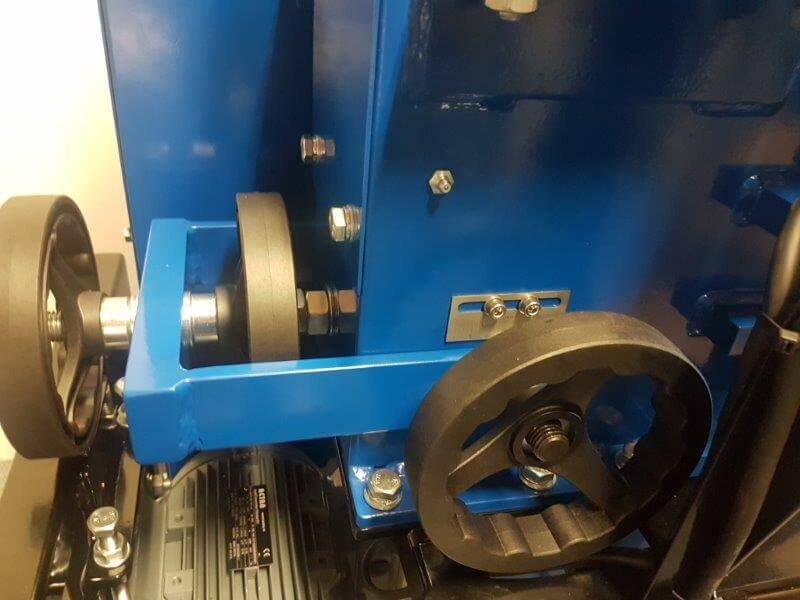
Hover
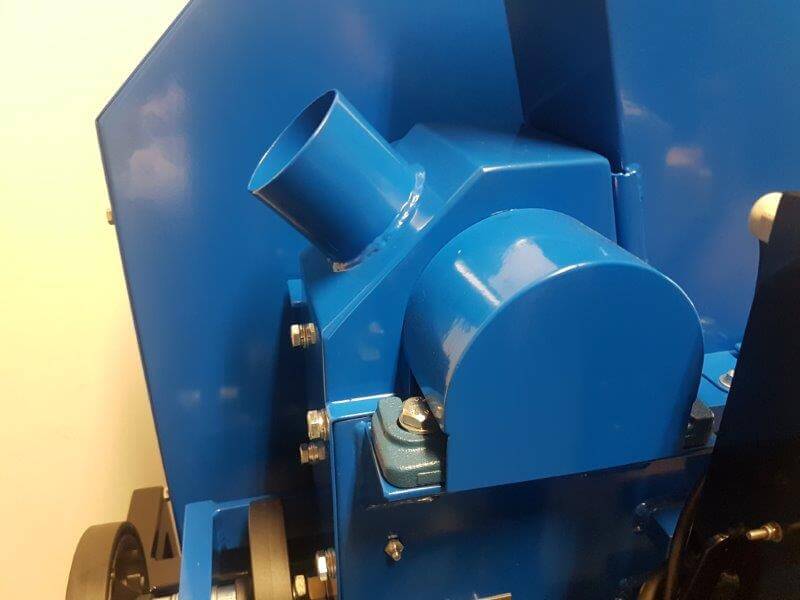
Hover
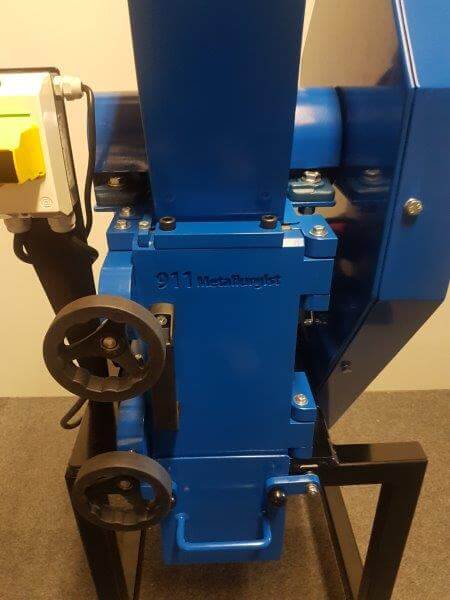
Hover
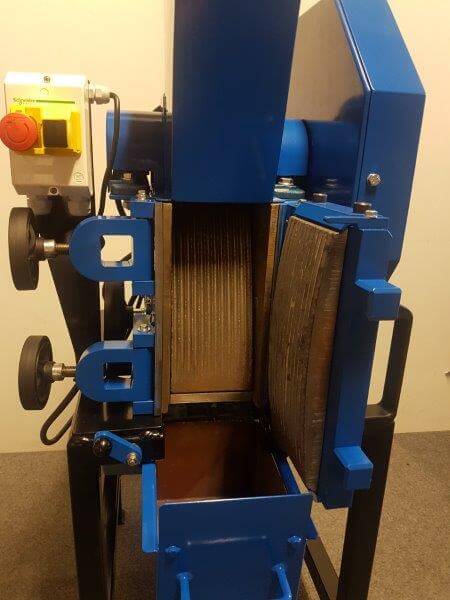
Hover
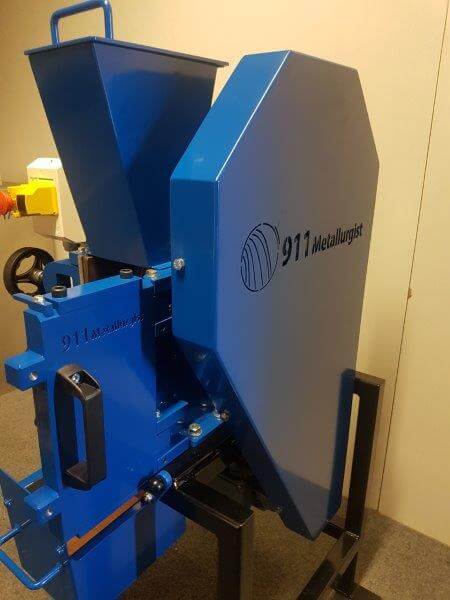
Hover
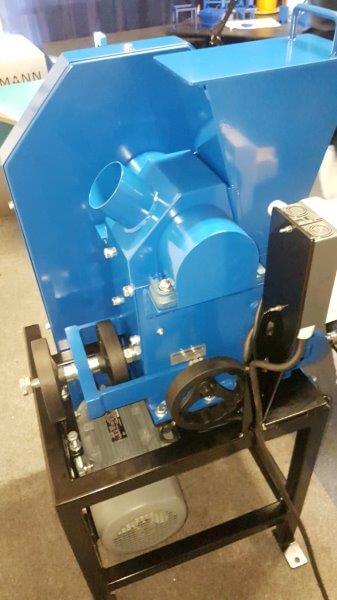
Hover
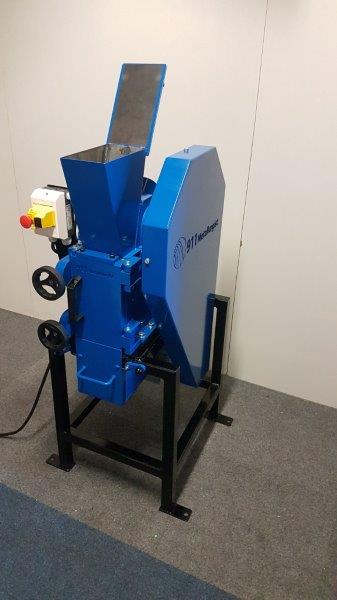
Hover
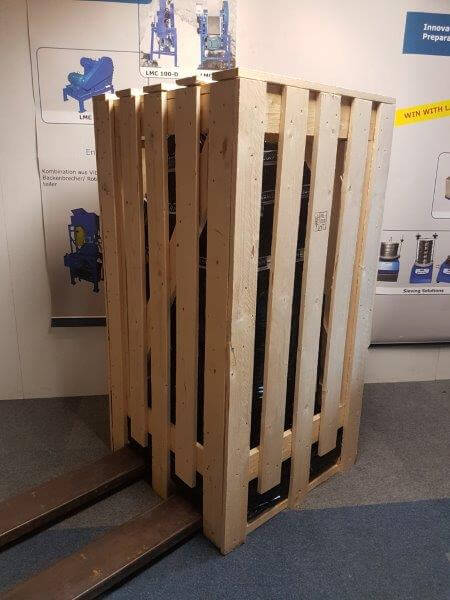
Hover
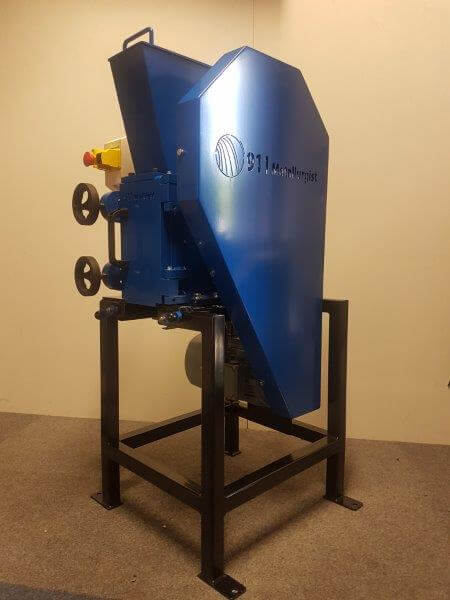
Hover
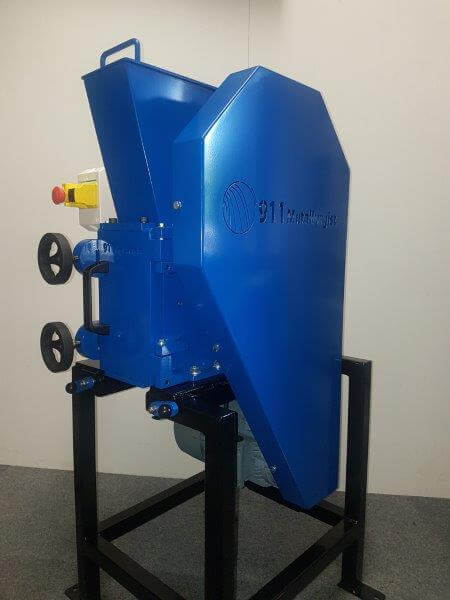
Hover
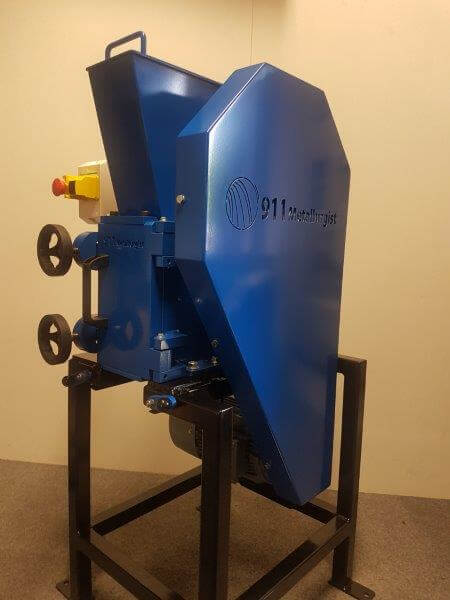
Hover
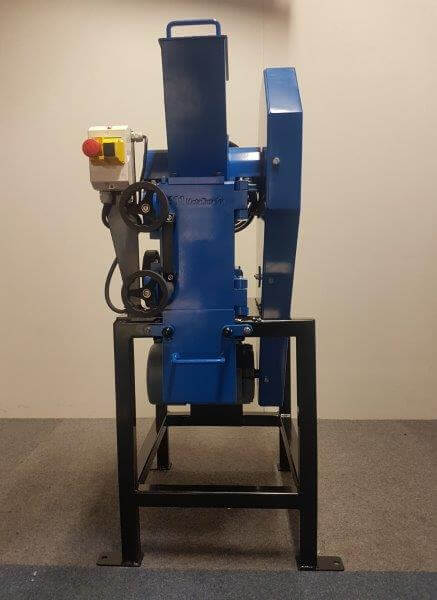
Hover
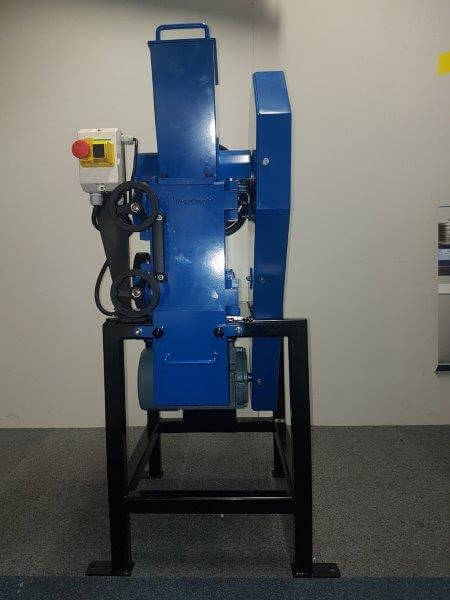
Hover
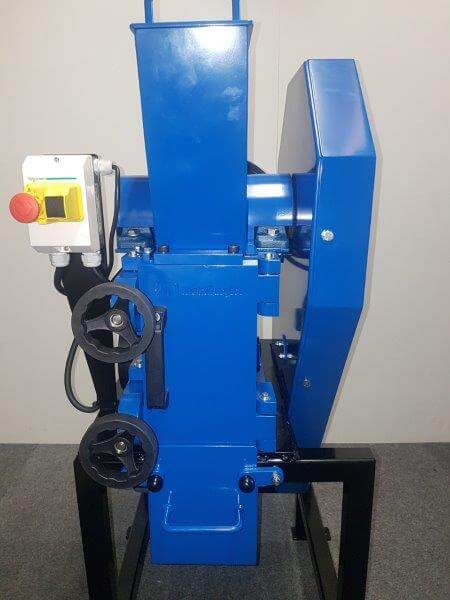
Hover
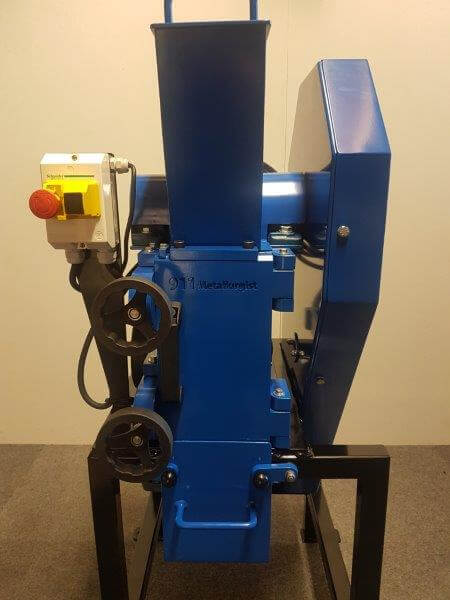
Hover
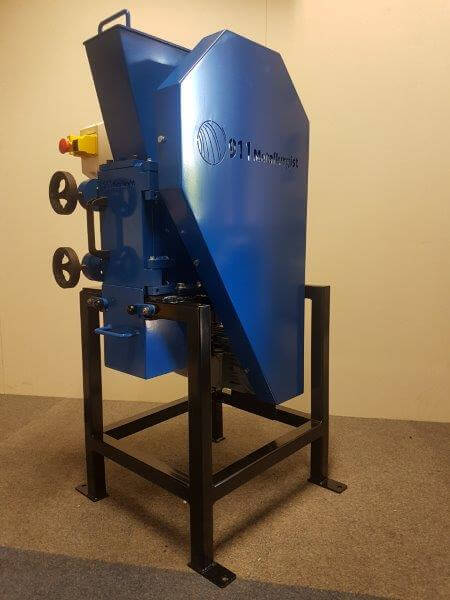
Hover
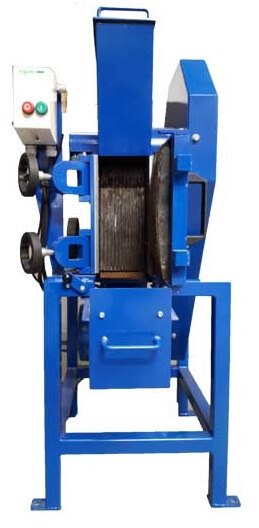
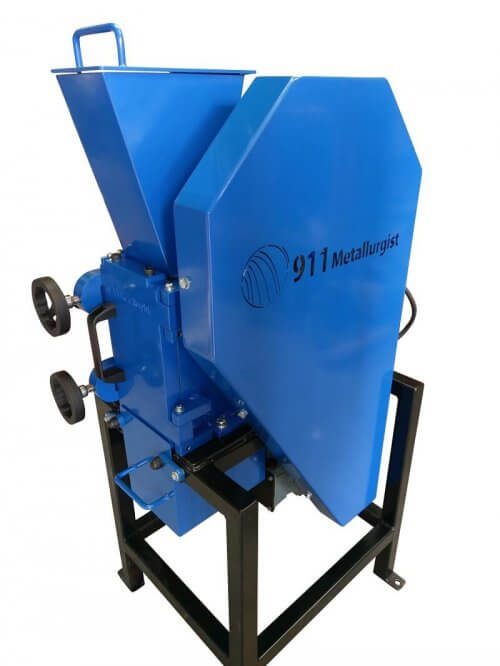
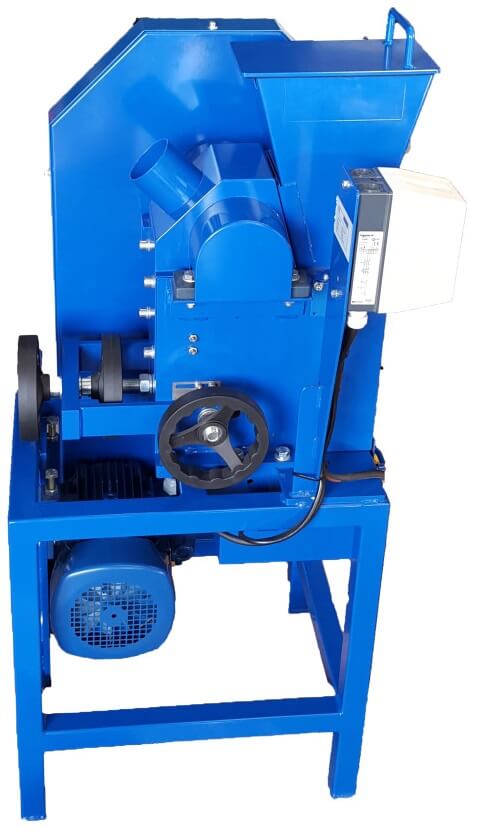
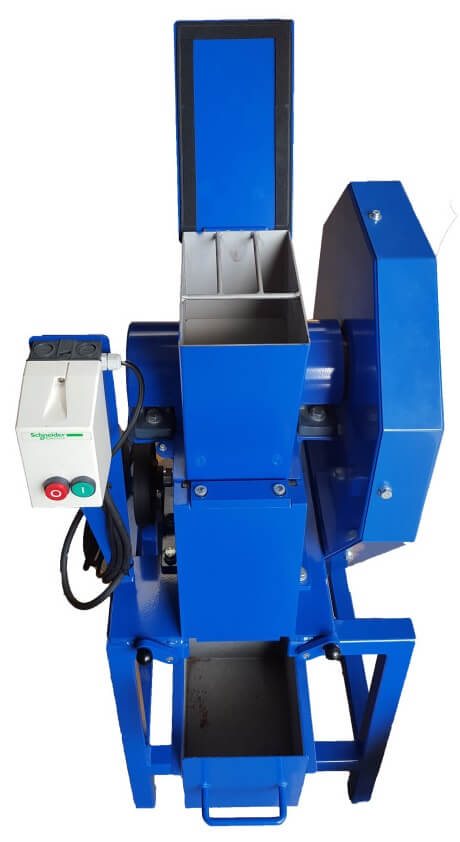
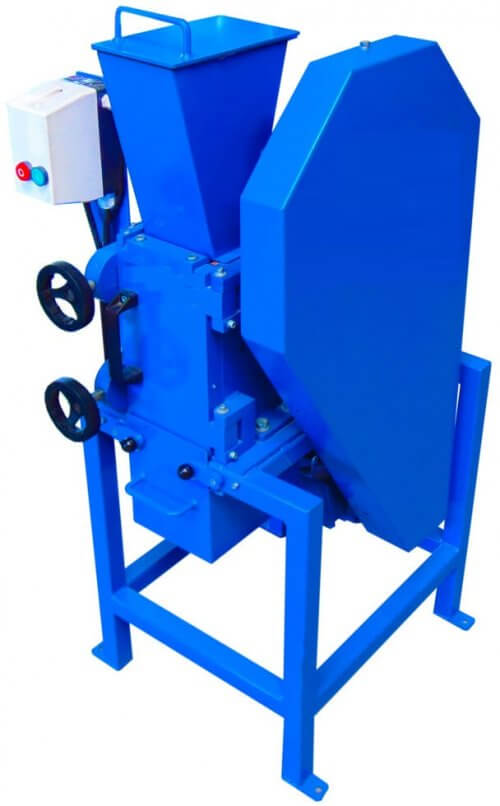
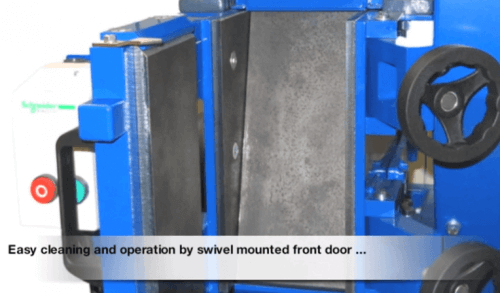
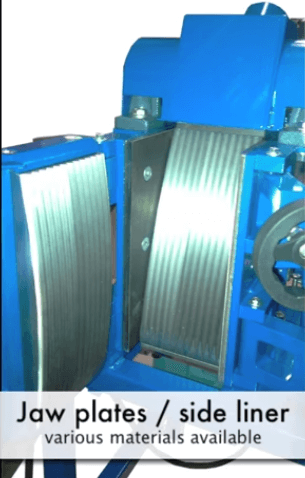
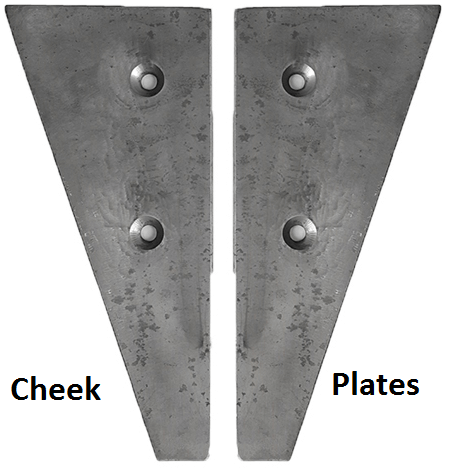
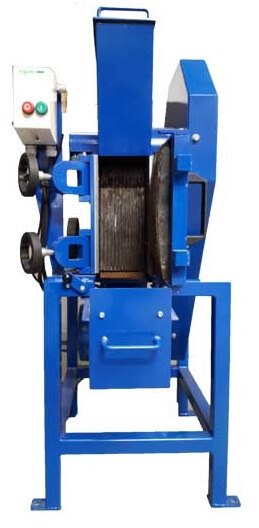
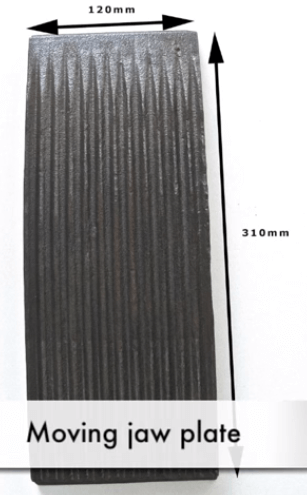
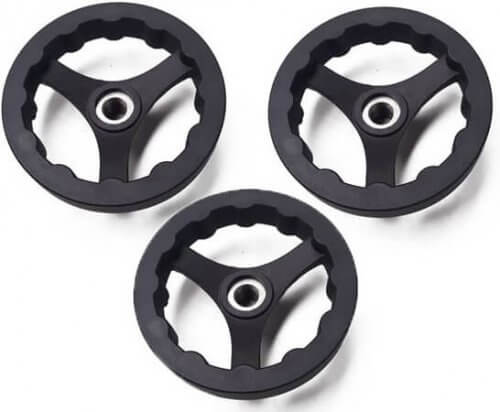
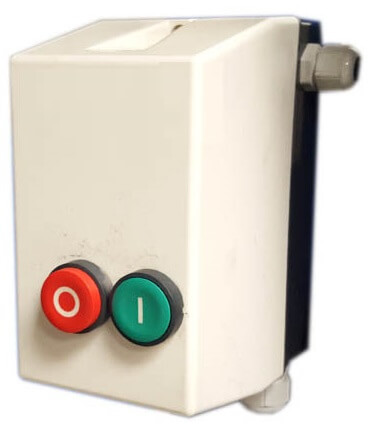
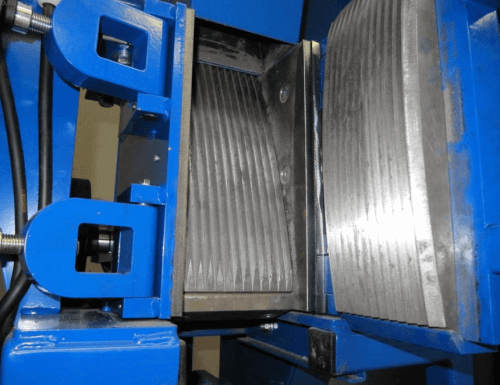
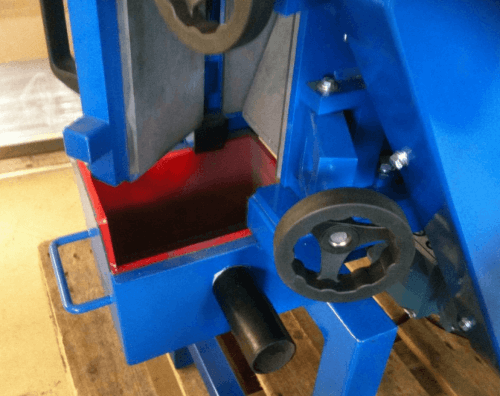
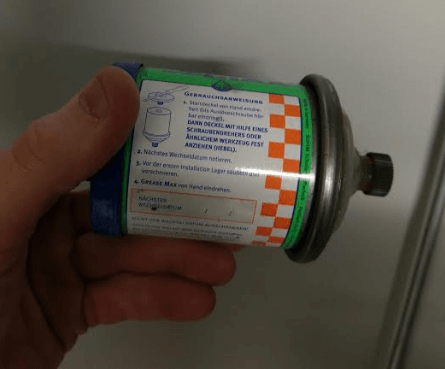
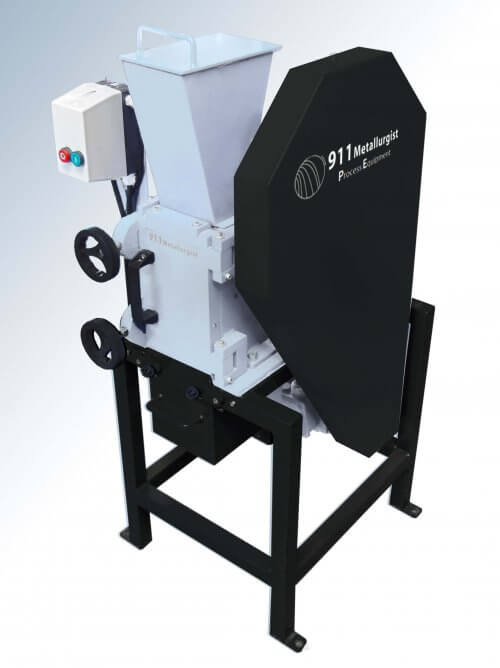
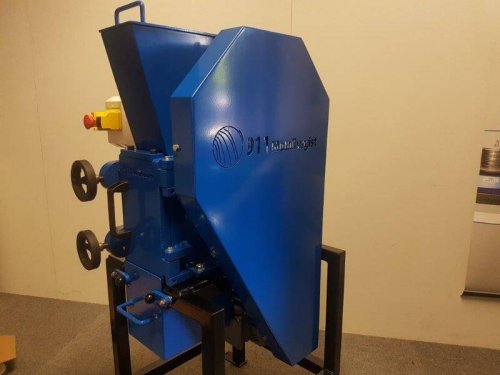

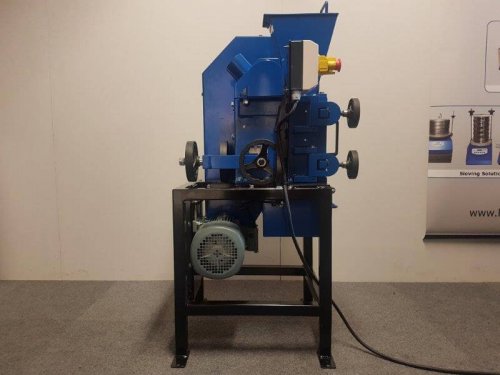
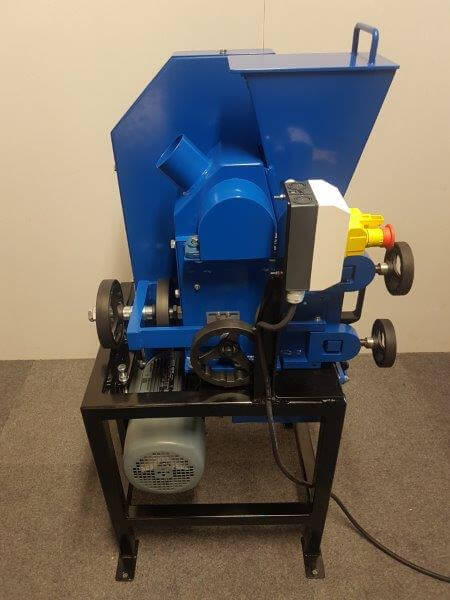
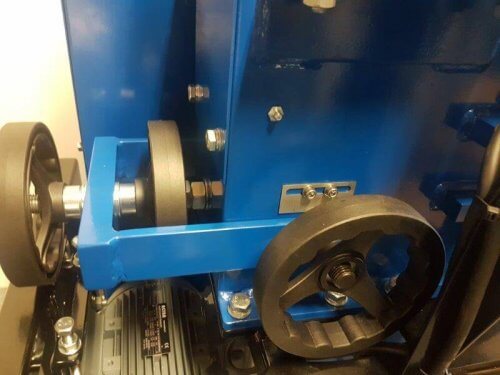
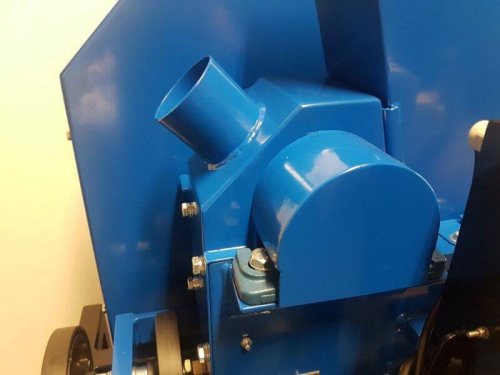
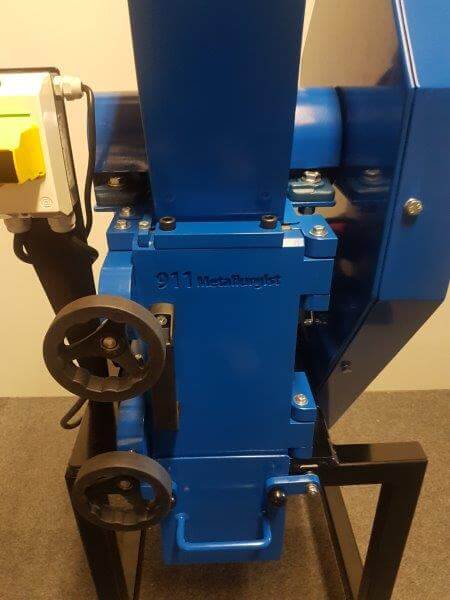
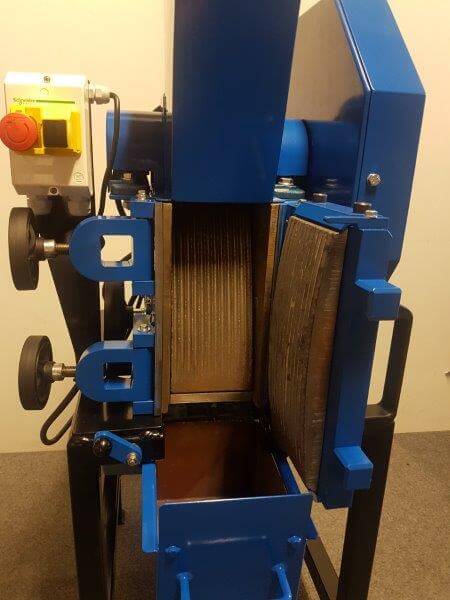
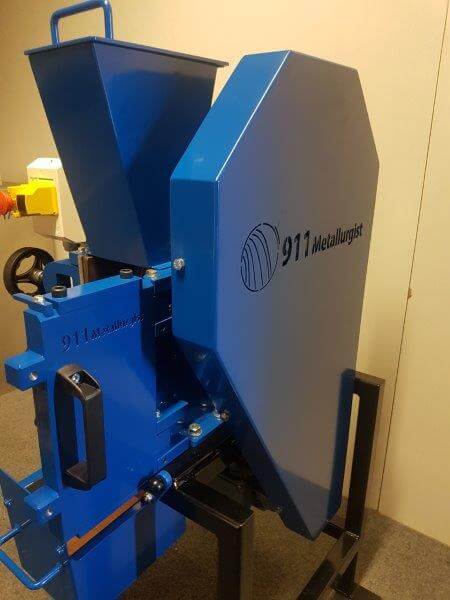
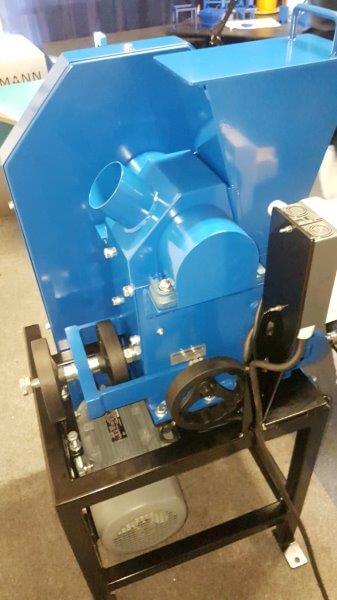
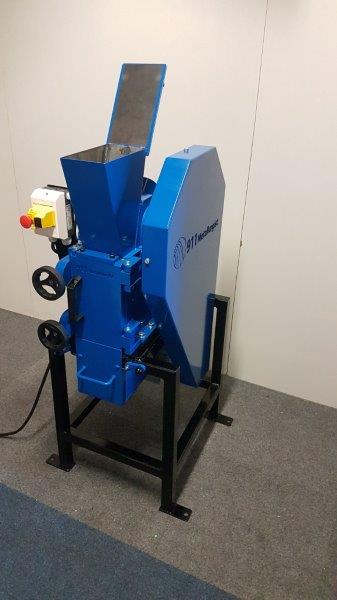
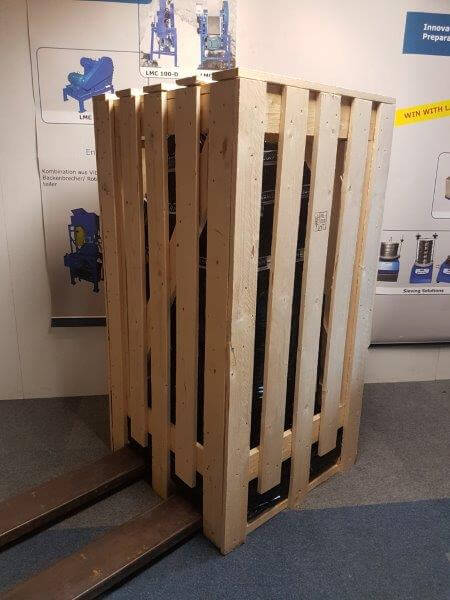
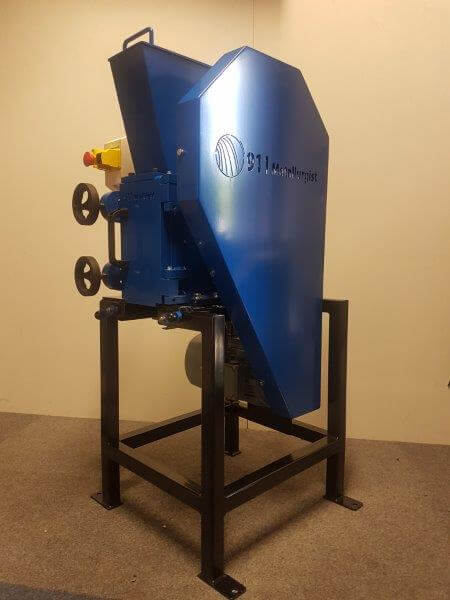
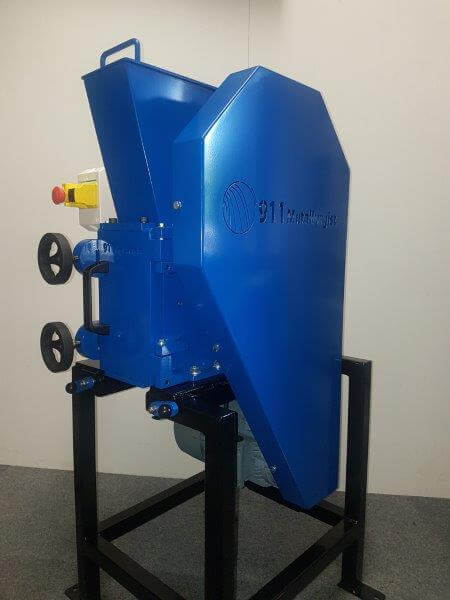
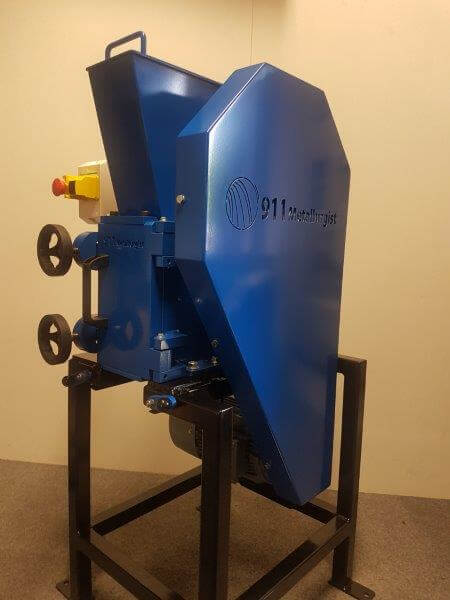
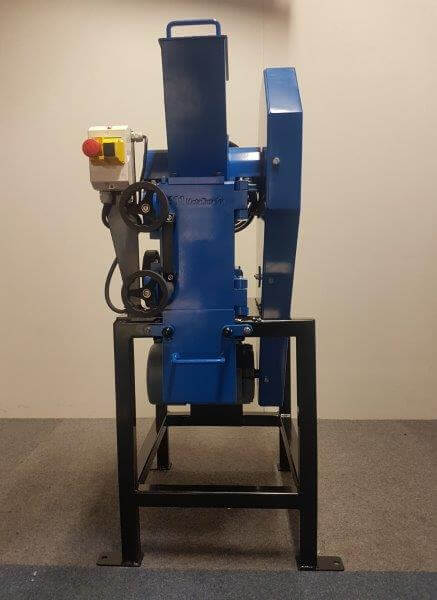
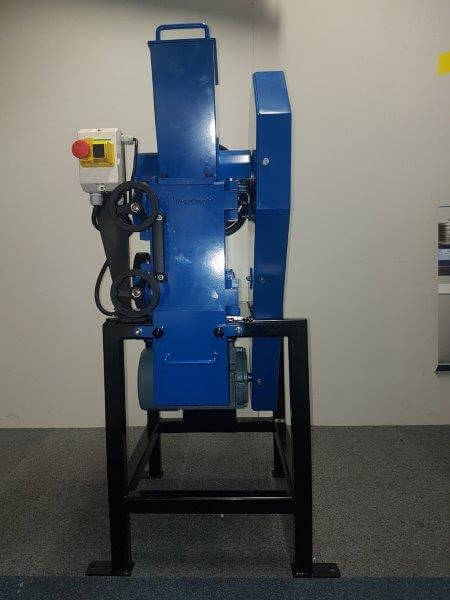
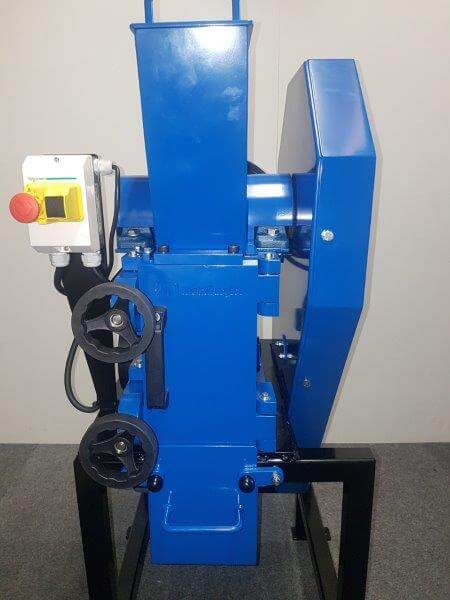
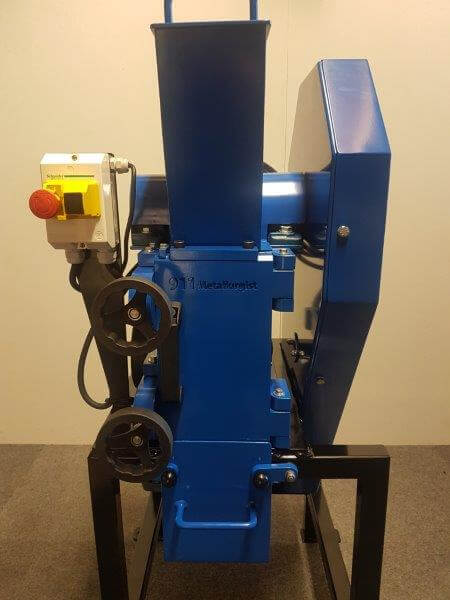
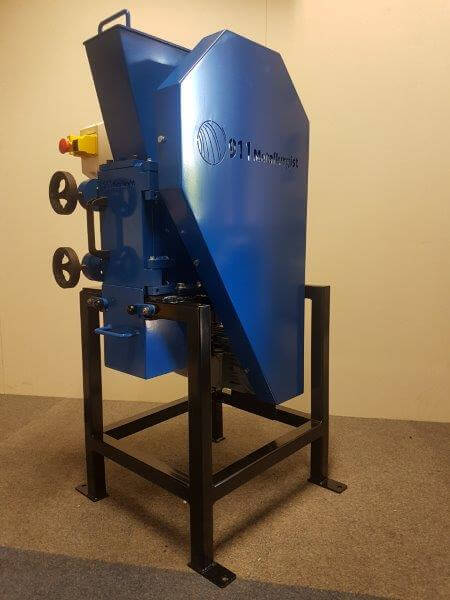
4″ x 5″ Laboratory Jaw Crusher 911MPEJC100
The 911MPEJC100-M/T Laboratory Jaw Crusher is engineered for rapid reduction of hard and brittle materials.
- 4″ X 5″ (100 mm X 130 mm) Jaw Opening
- Quick and easy to clean due to front door access
- Stepless, reproducible gap-setting by hand-wheel
- Manganese Jaw plates
- Easy exchange of jaw plates and side liners
- Modular infeed hopper: removable, with splash back protection, batch-loader or dosing plunger
- External control box with start/stop
- P80: < 2 mm
- Standard 3 x 380 V/50Hz Motor (customizable)
- Contamination-free crushing because of 4 different grinding tools
- This Crusher will crush
Minerals / ores / slags
Cement / cement clinker / concrete
Glass / ceramics / corundum
Soil samples / sludges
Coal / coke / drill core
Industrial waste
A jaw crusher robustly constructed single-toggle type with one fixed jaw plate and one moving jaw plate. Designed for the smaller laboratory, or use by prospectors. It is capable of quickly crushing materials up to 85% of the jaw opening (125 mm x 100 mm). It is robust and long wearing and made of parts and materials easily serviced in a remote area. Toggle adjustment is by means of jacking screw.
The crusher is supplied as a free standing floor mounted unit with the motor to the rear of the main body.
Comes complete with dust extraction point (from the top of the machine), hinged inlet hopper and removable sample bucket.

Description
A Laboratory Jaw Crusher engineered for pre-crushing of extremely hard up to brittle materials. The 4″ x 5″ 911MPEJC100 Jaw Crusher is designed for batch and continuous crushing of middle hard, hard brittle and tough materials for the following fine grinding.
Principle of operation of this crusher
The Model 100 mm X 130 mm 911MPE-JC100 Jaw Crusher is used by laboratories and processing companies to crush solid materials such as rocks or soil and ores. The material to be processed falls into the crushing chamber of the 911MPE-JC100 Crusher via a guide chute and is crushed by crushing action of a static and a dynamic jaw plate until it passes the preselected gap of the crusher. The stepless gap setting can be adjusted via a hand wheel and a mm scale from 0 mm to 25 mm (contact of the jaw plates).
This adjustment setting enables the operator to also perform a zero point calibration setting to adjust the crusher.
Various infeed hoppers made of stainless steel or coated with PU are optionally available.
Easy cleaning and operation
No other Laboratory Jaw Crusher is easier to clean than the 911MPE-JC100Jaw Crusher. When the crushing process is finished the infeed hopper can be taken off in a second and the front door opened. Due to these points the crushing chamber is fully accessible for quick and easy cleaning in order to perform a fast and systematic cleaning of the grinding tools.
Jaw plates / side liners material compositions
![]() |
| ![]() |
Crusher Gap adjustment
The required gap setting can be comfortably and reproducibly adjusted by rotating the handwheel. The gap setting can therefore steplessly pre- chosen according to a scale prior to start the crushing process.
Maximum Feed Size | 115 x 90 mm |
End fineness | <2 mm |
Approximate Mass | 250 Kg |
Approximate Throughput | 25 to 50 Kg/hr (hardness + feed size + product size dependent) |
Dust extraction points | 1 |
Dust Extraction flow | 150 L/sec. |
Adjustment of Crushing time | Start/Stop |
Electr. requirements (3-Phase) | 4 HP |
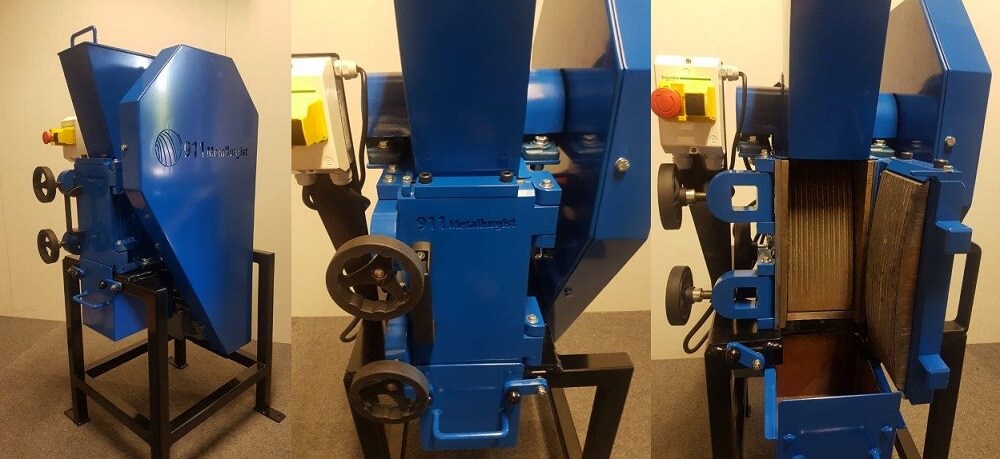
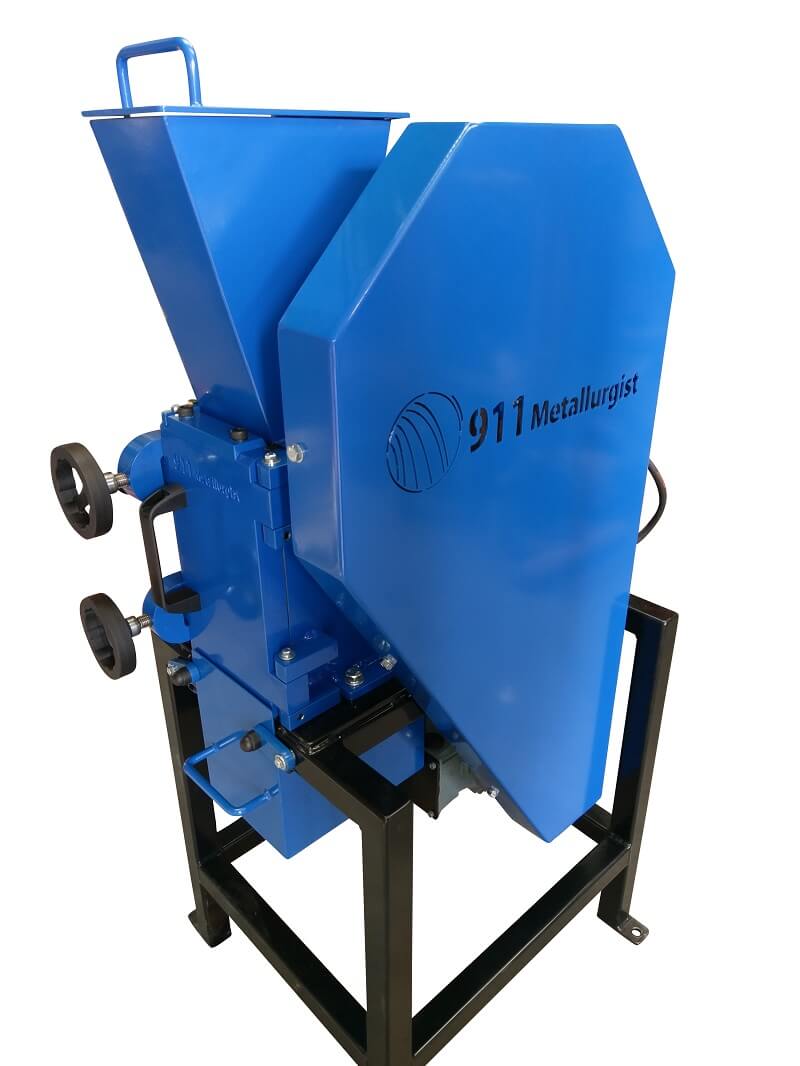
This crusher, which lies within the family of jaw crushers. It is a floor-standing instrument as we can see, mounted on a very sturdy mounting frame. The 911MPE-JC100 can accept pieces of material in the top feed hopper with a maximum of 120*90 mm and reduce them in size to less than 2mm often in a single pass. This is a very wide reduction ratio commonly used in heavy industry applications. Jaw crushers such as the 911MPE-JC100 model are used for the preparation of hard, medium-hard, brittle, and tough materials. Common examples of application areas would include the preparation of construction materials, rocks, cement, metallurgy, and many more. Supplied with the instrument is a 10-liter collecting receptacle to collect the crushed material, it is positioned ergonomically at a convenient working height. A further optional accessory is a second collecting receptacle for continuous processing. The sample material is crushed between the fixed and the moving break in the jaws, after the crushing has taken place it is important to clean the jaws thoroughly to prevent any cross-contamination of the samples. The instrument is fully safety interlocked, you can simply remove the collecting receptacle and the front door is opened as it is hinged then we have complete access to the crushing chamber and the jaws for cleaning purposes which is very convenient. On the rear of the instrument, we see a standard size dust extraction port that will be compatible for use with most dust extraction systems. Also available is an adapter if the client wishes to use an industrial vacuum cleaner. This instrument is designed with convenience in mind therefore with the use of a standard single Allen key we can remove the Allen screw and simply lift and remove the feed hopper for cleaning purposes. Operation of the instrument couldn’t be easier; we simply depress the start button. Importantly the gap between the fixed and moving jaws is steplessly adjustable; this can be done using the scale from the range of 0-30mm. I trust that you found the overview of the 911MPE-JC100 to be very useful.
Jaw Crusher Features and Benefits
- Better crushing performance due to 3000 Watt (strong 3 phase motor)
- Optimum crushing results
- Easy and quick access to the crushing chamber (no tool required)
- Stepless adjustment of gap setting (no tool required)
- CE certified
- Removable infeed hopper with safety baffles or batch loader
- High precision solid steel underframe (available with lockable castor wheels)
- Solid and spacious 10 liter sample collector
- Motor protection switch with start/stop
- End fineness P80 < 2 mm
- Contamination free crushing because of 4 different grinding tools*
- Easy exchange of jaw plates and side liners
Crushed Material Examples | |
![]() | ![]() |
![]() | ![]() |
![]() | ![]() |
![]() | ![]() |
Jaw Crusher 911JC100 | |
complete with door, feed hopper, collecting drawer & base frame | |
Jaw Crusher Liner Plate | Side Cheek Plates |
manganese steel | hardened steel |
tungsten carbide | hardened steel |
tungsten carbide | tungsten carbide |
steel absent of Heavy-metals | steel absent of Heavy-metals |
Optional Separation Unit | |
Special version with an automated sorting machine with 3 fractions for undersize and 1 fraction for oversize (230 V/50 Hz). | |
other electrical versions available for the same price | |
Accessories | |
Collecting drawer, standard steel, powder-coated, 5 liters | |
Collecting drawer, standard steel, powder-coated, 10 liters | |
Collecting drawer, stainless steel, 30 liters (drum) -connection flange and carrier with wheels | |
Collecting funnel with an outlet for continuous use, powder-coated | |
Infeed hopper, stainless steel, 10 liters -includes connection rod & manual pusher | |
Automatic Lubrication | |
Automated sorting machine with 3 fractions for undersize & 1 fraction for oversize (230 V/50 Hz) | |
Suggested Spare Parts | |
Jaw Crusher Liner Plate | Side Cheek Plates |
![]() | ![]() |
![]() | ![]() |
NEW SPECIFICATION of the JAW CRUSHERS
A new 20-page Specification Manual describing the complete line of Jaw Crushers is now available. Jaw Crushers are available in 21 different sizes and capacities from the 2¼” x 3½” Laboratory unit to the large, split-frame 40″ x 48″ type “J” giant, as well as in various portable and semi-portable crushing plants. In addition to dimensions, weights, power requirements, material specifications, and capacities for all Jaw Crushers, this manual illustrates in cut-away style the bearing design.
Jaw Crushers are of “all anti-friction bearing” design. The side bearing of the larger type “H” Crushers have the anti-friction side bearing housed in a self-aligning ball-and-socket type carrier. This makes it possible to remove the main shaft without ever exposing the bearings to dust or dirt.
Crushers have steel frames and feature genuine 13-14% manganese steel jaw plates. Most sizes are maintained in stock for quick shipment.
Every engineering library should have one of these new manuals, and everyone contemplating the purchase of a Jaw Crusher should first compare the specifications and price of Crushers.
Rock Crushers are used to obviously, crush big rocks into little rocks. Since we are small-scale process experts, we offer mini, small to ‘not so small’ rock crushers categorized as the jaw, cone, hammer, and roll. Most are small enough to use in a home-style laboratory or any full commercial-industrial laboratory, while the largest unit equipment adequately processes all your needs for small-scale mining equipment.
For the crusher to correctly perform its role in the processing cycle with maximum efficiency and economy it must be matched to the final task. Determining factors in crusher selection can be broken down into four categories: material to be crushed; feed size; product size; and expected capacity. The right crusher should also have the lowest power requirements per ton of finished product, and operate with minimum maintenance and downtime. There are a variety of crushers to meet the needs of today’s industrial requirements. Exactly how is a determination made on the proper crusher? Usually, extensive study and evaluation of the above facts concerning material, feed size, feed rate, capital cost requirement, etc. will be an essential guideline. Next to be considered is the actual mechanical method of crushing to be used.
It is our approach that determines 911MPE product’s to be reliable and superior and makes our jaw crushers ideal for coarse and initial size reduction of hard to brittle samples. The reliability of the 911MPE jaw crushers is based on their extremely robust design. Thousands of installed crushers all over the world within the last decades demonstrate the trust of many customers. Their superiority is represented by two decisive competitive advantages, the large number of technical highlights as well as the unrivaled selection of crushers and breaking jaws that are developed to suit the requirements for different feed sizes, materials, and applications. 911MPE jaw crushers are available in four different models starting from the benchtop model and ranging up to the strongest floor model. Our machines belong to the family of single toggle jaw crushers; a high final fineness and excellent crushing ratio demonstrate their great efficiency. The main fields of application include construction materials, mineralogy, and metallurgy, ceramics and glass materials as well as research and environmental analysis.
Jaw crushers are particularly suitable for the preparation of rocks, minerals, ores, glass, ceramics, slags, synthetic resins, and many hard brittle substances. Depending on the model samples of up to 150mm in feed size can be ground to a final fineness of down to less than 0.5mm. For a further introduction of our range of jaw crushers, we are going to focus on the widely established JC100 which is the core product of our floor models and suitable for many major applications. After starting the machine the gap width is set by using an analog scale it ensures optimal size reduction in accordance with the desired material fineness. The sample can then be filled into the no rebound hopper and enters the crushing chamber, size reduction takes place in the wedged shape area between the fixed crushing arm and the one moved by an eccentric drive shaft. The movable jaw is driven by a heavy-duty brake motor via v-belts; the largest belt pulley also acts as a flywheel to ensure uniform and smooth operation. The elliptical motion crushes the sample successively as soon as the sample is smaller than the gap width, it falls into a removable, easy to clean, stainless steel collector. By using state-of-the-art modulation methods during the design process of our crushers we ensure to archive the best performances. Due to the optimized eccentric movement and the special geometry of the grinding chamber, the 911MPE JC100 achieves a much higher fineness than traditional jaw crushers.
The grinding chamber is dust-protected using a commercially available vacuum cleaner; additional dust extraction can be achieved without affecting the result. Depending on the material in the throughput sooner or later even high-quality breaking jaws will start to show signs of wear, this means that the set crushing gap will increase with time. In order to still be able to obtain reproduce-able crushing results, this wear must be compensated with traditional jaw crushers whose gap width can only be set in fixed steps and accurate compensation for wear is not possible. 911MPE jaw crushers can be continuously adjusted and therefore the wear of breaking jaws can be compensated precisely. We provide user convenience combined with maximum safety. Compared to traditional jaw crushers the crushing chamber is accessible via quick release clamps, this enables easy cleaning and exchanging of the breaking jaws. An important safety aspect is provided by a switch that interacts with a brake motor to ensure immediate stops if the unit is opened during operation. A further safety feature is that the hopper is equipped with sample rebound protection which also ensures that the crushing chamber can’t be accessed by hand; a disc spring unit and thermal overload protection prevent abuse of the system. Like all 911MPE machines, the JC100 is designed to be in full compliance with the highest safety regulations. 911MPE jaw crushers run smoothly and are surprisingly quiet, due to their robust design they are not only applicable for batch-wise operation but also particularly suitable for continuous size reduction in online operation.
Optional kits for the central lubrication of the lower movable crushing arm are available as well. This is particularly advantageous if the jaw crushers are to be used continuously or if they are installed in a cabinet in such a way that the lubrication points are difficult to access. Because of their solid design they are virtually maintenance-free and perform their size reduction duties over a long lifetime. Our floor-based crushers as presented so far are complemented by the benchtop alternative which has been specifically designed for sample preparation of small laboratory quantities. The space-saving dust-type instrument fits on any lab bench. Small amounts of samples with feed sizes of up to 35mm are crushed gently and without any sample loss. Breaking Jaws for the entire jaw crusher range providing excellent surface quality for the highest demands. Jaws and side plates are available in various materials such as manganese steel which is made from precision casting, tungsten carbide, stainless steel, heavy metal free steel, and zirconium oxide. Thus versatile applications can be fulfilled without cross contaminations. 911MPE crushers are the ideal choice when the rapid and gentle size reduction of hard and brittle materials is to be accomplished. Due to the optimized movement the favorable geometry of the grinding chamber and the wide variety of grinding materials, excellent grinding results are achieved which looks after all requirements. The reliability and superiority of our products make 911MPE the leader in crushing and pre-crushing for sample preparation. Thousands of installed units all around the world demonstrate the trust of many customers.
Crusher Bearings & Lubrication
Please look at the pictures of the bearings at the top of the machine. As you can see they are on the outside of the crushing chamber.
The bearings at the bottom of the movable plate are made from brass. The bearings are not the standard ball bearings as on the top of the machine, but tubes made from brass. The picture on the attached file shows the brass tubes. The lubrication of this bearing is very important and can be done by a lubrication cartridge.
The cartridge will be activated using a “chemical switch”. The switch will press the greasing out of the cartridge and can be set on a certain “empty time” from 1 to 12 months. When the greasing level in the bearing is good, the dust will be pressed out preventing the bearing from locking/breaking. Picture attached from the position the cartridge will be placed as well as the connections inside at the messing bearing.
Additionally:
Easy access door for maintenance and cleaning. When the operator spends a few minutes after each run or after each day cleaning the machine the lifetime will expand dramatically. Because our machine is not closed and therefore easily accessible cleaning and maintenance can be done properly.
The gap settings a stepless. Operators only need to untighten one wheel on the side and set the gap using the other two wheels. When the jaw plates wear, the scale on the side of the machine can be adjusted in order to receive the new 0-point.
The hopper on top of the machine can be made according to your requirements. We can build an infeed hopper to hold a 5-gallon “Bucket holder” at 45 degrees while it flows into the crusher cavity.
Laboratory Rock Crusher
The 4″ x 5″ is a Laboratory Rock Crusher engineered for rapid reduction of hard and brittle materials. The 911MPCJC200D Jaw Crusher is designed for batch-wise and continuous pre-crushing of middle hard, hard brittle, and tough materials for the following fine grinding.
- Quick and easy to clean due to front door access
- Stepless, reproducible gap-setting by hand-wheel
- Easy exchange of jaw plates and side liners
- Modular in-feed hopper: removable, with splashback protection, batch loader or dosing plunger
- External control box with start/stop
- End-fineness: < 4 mm
- Contamination-free crushing because of 4 different grinding tools
Principle of operation
The Model 911MPCJC200D Jaw Crusher is used by laboratories and processing companies to crush solid materials such as rocks or soil and ores. The material to be processed falls into the crushing chamber of the 911MPCJC200D Crusher via a guide chute and is crushed by the crushing action of a static and dynamic jaw plate until it passes the preselected gap of the crusher. The stepless gap setting can be adjusted via a hand wheel and a mm scale from 0 mm to 25 mm (contact of the jaw plates). This adjustment setting enables the operator as well to perform a zero point calibration setting to adjust the crusher.
The 911MPEJC200 Jaw Crushers for Coarse Primary Crushing and Mid-Range Primary Crushing. Its 3.5″ X 8″ jaw cavity and 7.5 HP motor crush up to 1 TPH down to a P80 of 5 mesh.
A well-proven single toggle, high reduction rock crushers. The crushing capacity and the end-fineness of the sample material depend on the type of crusher and on the breaking characteristics of the sample material. Designed for laboratory applications and for operating environments and accepts:
- soft to hard ores
- drill cores
- rocks
- alloys
- slags
- soils
- coal/coke
- cement
- granite
- ceramics and similar hard/brittle materials
- Industrial waste
| Jaw plates / side liners material compositions • Hardened steel • Tungsten carbide • Heavy metal-free steel • Stainless steel |
Easy cleaning and operation
No other Jaw Crusher is easier to clean than the 911MPCJC200 Jaw Crusher. When the crushing process is finished the infeed hopper can be taken off in a second and the front door can be opened. Due to these points, the crushing chamber is fully accessible for quick and easy cleaning in order to perform a fast and systematic cleaning of the grinding tools.
• Optimum crushing results
• Easy and quick access to the crushing chamber (no tool required)
• Stepless adjustment of gap setting (no tool required)
• CE certified
• Removable infeed hopper with safety baffles or batch loader
• High precision solid steel under-frame (available with castor wheels lockable)
• Solid and spacious 10 liter sample collector
• Motor protection switch with start/stop
• End-fineness < 4 mm
• Contamination free crushing because of 4 different grinding tools*
• Easy exchange of jaw plates and side liners
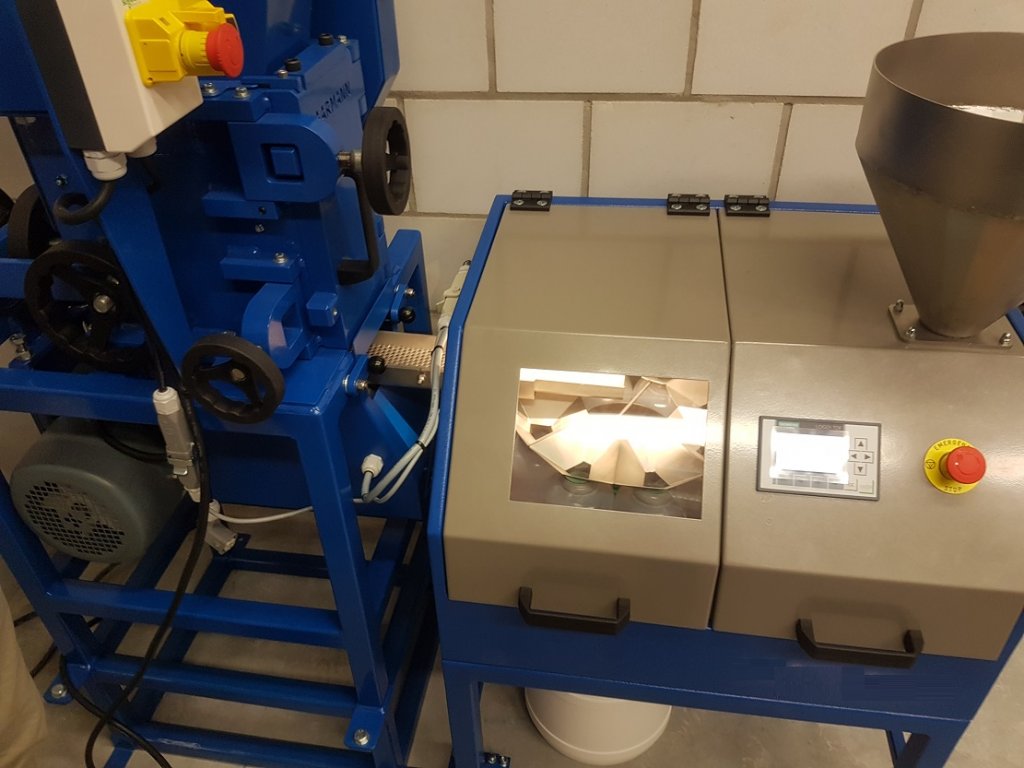
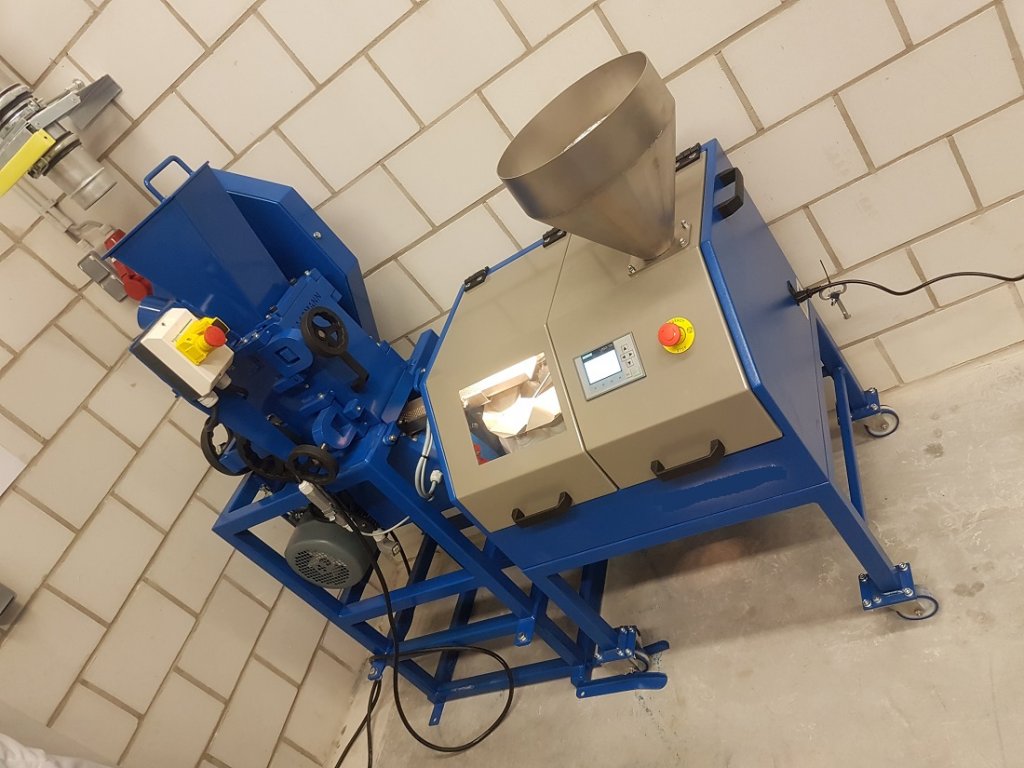
Crusher Liner to Avoid Metal Contamination
| ![]() |
|
|
Laboratory Jaw Crusher
In these crushers one jaw is fixed and the other is swung back and forth, through a very small arc, by means of an eccentric shaft, revolved by either the hand wheel or the pulley. The shaft in revolving raises and lowers a rod which is connected by toggles with the movable jaw. These crushers can be procured in sizes ranging from laboratory size up.
The Bosworth Crusher has been much improved upon of late, particularly with respect to the ease with which it can be adjusted and cleaned. Below will be found mention of three of these crushers.
The Case Laboratory Crusher (patented in 1903), Fig. 69, was especially designed to meet the demand for a low priced, strong, laboratory crusher. It can be driven by either hand or power, and will crush from 100 to 200 lbs. of ore per hour, depending, of course, on the hardness of the ore. The jaw opening is 2½ by 3 inches and it can be adjusted quickly from a fineness of ¼ inch to 20 mesh. The feed is regular, and it is not inclined to cake on soft material. Messrs. E. H. Sargent & Co., Chicago, handle this crusher which they sell at $30.00, hand driven, and $32.00, fixed for power.
Figure 70 shows the Calkins Advance Ore Crusher, manufactured by The Calkins Company, Denver, Colo. One of the features of this crusher is the ease with which it can be cleaned. This is done by swinging up the front jaw of the crusher exposing the sides and face of the vibratory jaw and giving access to all parts of the machine to which material being crushed may adhere. The adjustment to coarse or fine crushing is made at the front end of the crusher. This machine is made in two sizes; the smallest has a jaw opening 2½ x 3 inches, weighs 170 pounds, and is listed at $30.00 net; the larger size has a jaw opening 3 x 4 inches, weighs 280 pounds and is listed at $60.00 net.
The “Lightning” Crusher, Fig. 71, manufactured by F. W. Baun & Co., Los Angeles, Cal., is also a very efficient crusher
which may be adjusted to crush fine or coarse. This crusher is also very easily cleaned, as, after lifting a pin, the front jaw may be swung out on a vertical hinge exposing both crushing plates and allowing them to be quickly and thoroughly cleaned with a brush. This crusher is very compactly and strongly built.
Where very large quantities of ore have to be crushed a Gates gyratory crusher will be found most useful. These are made by the Allis-Chalmers Co., Milwaukee, Wis., in a variety of sizes and types. The smallest size will crush 500 lbs. of material of average hardness per hour, to a fineness of ¾ inch or 250 lbs. to 1/8 inch.