Table of Contents
The scope of this paper is the description of two districts in Peru in which vanadium deposits have been found, and the consideration of much laboratory-work that I and others have done to determine the nature of these deposits. It is with regret that I present some of the data in a state of evident incompletion, particularly the analyses of a few of the minerals, which leaves some doubt as to whether the true nature of the minerals is understood. I make no apology, however, since in this case, as in many others referring to ore-deposits, conclusive opinions cannot be stated until a great amount of work has been done, and the deposit has been thoroughly exploited. I believe that the data and the tentative conclusions here presented indicate a unique and interesting condition which may assist others in work along similar lines; and further, what is of more practical importance, stimulate a search for a metal which, though long considered rare, is probably so merely because proper care has not been taken to recognize it.
An excellent bibliography on the occurrence of vanadium in nature is given by F. W. Clarke in his paper, Data of Geo-chemistry; and in the preparation of this paper I have consulted many of the references contained in the Bibliography of the Chemistry of Vanadium, by M. Moissan. A brief review of the subject having special reference to the metallurgy of vanadium is contained in the small book by P. Nicolardot.
One of these vanadium-deposits has already been briefly described, and analyses of some of the minerals have been published.
Both of the vanadium districts are situated in the Department of Junin; Yauli, the less important, being in the Province of Tarma, and Quisque (Minasragra) in the Province of Pasco. The Peruvian Central railroad (Ferrocarril Central Del Peru) passes through the first district, and the second is accessible by
horseback from Fundicion on the Cerro de Pasco railroad, as shown in the map, Fig. 1.
Though within 80 miles of the Pacific ocean, both districts are on the eastern slope of the main range of the Andes, and the drainage in each case is into the Mantaro river, the waters of which enter the Ucayali, the main branch of the Amazon.
Topographically, the districts are similar, though the relief in the Yauli region is stronger. The features depend upon geological structure rather than variety of rock; faults and folds in the sedimentary rocks being common.
The main range of the mountains, lying approximately N. 30° W., is well defined. Summits often attain elevations of 18,500 ft., and passes are seldom lower than 16,000 ft. The main range is succeeded to the east by similar though lower parallel ranges. The intervening valleys, when narrow, resemble the U-shaped glacial valleys of the Rocky mountains, but when broad (up to 20 miles) they are low in relief, and contain many lakes and marshes.
There are two distinct seasons—the wet, which begins during November and lasts until May; and the dry, which comprises the remaining months. During the wet season precipitation occurs daily and regularly, even to the hour. Above 14,000 ft., in latitude S. 12°, it is invariably snow, and is often confined to the higher ranges. Except in sheltered places, the snow melts quickly below 17,000 ft., so that it can be understood that erosion by water in altitudes of from 14,000 to 17,000 ft. is a minor feature, a consideration that has great bearing upon the deposit in the Quisque district.
During the dry season precipitation may occur, but it is irregular, and streams in the upper valleys become dry to such an extent, in fact, that the herds of sheep and llamas must he taken to the lower valleys for pasture. Severe electrical storms, unaccompanied by rain, are common during the dry season.
A description of the Yauli district will precede that of the more important Quisque district, for the reason that the first enables a more thorough understanding of the second, as well as the fact that this was the order in which I examined them. It was a coincidence that the Quisque deposit was discovered while I was examining the Yauli district.
Yauli District Geology
Historical.—Though not announced until 1894, the presence of vanadium in the so-called “anthracite” (asphaltite) of the Yauli region was known in 1892. Theretofore, the asphaltite had been held under denouncement, and exploited with the view to utilizing it as fuel. In 1895 the Llacsacocha deposits were acquired by a French company, whose principal resources were some nearby silver-mines (Andaychagua). This company attempted to utilize the vanadium contained in the asphaltite, but, with the impoverishment of the silver-veins in depth, the company became bankrupt and ceased operations in 1899. It is reported that several tons of vanadiferous ashes were sent to France for treatment, but beyond this very little asphaltite was mined. No work has been done since 1899.
Several of the less-important deposits in the northern portion of the district have been developed to a slight extent, and the asphaltite locally used for fuel.
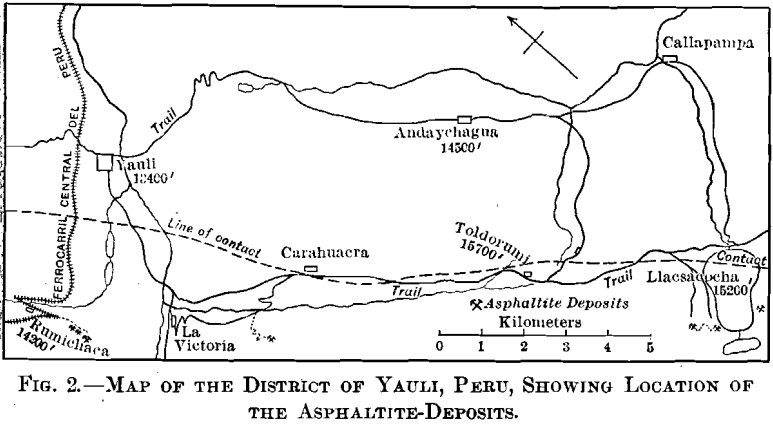
Geology
Fig. 2, embracing an area about 5 by 12 miles, gives the location of known occurrences of asphaltite.
The broken line on the map marks the contact between folded Jura-Trias and Cretaceous sedimentary rocks on the southwest, and more recent eruptive rocks on the northeast, which contain the silver-veins of Andaychagua and the copper-silver veins of Yauli. The famous Carahuacra silver-mine, and several less important, are in the contact-zone.
The Jura-Trias rocks are coarse conglomerates and sand-stones, and the Cretaceous is represented by thin-bedded gray and green shales and limestones, the latter predominating, illustrated in Fig. 14. In the vicinity of Toldorumi the intrusion of the eruptive rocks on the northeast has brought up a narrow belt of slates.
The veins of asphaltite occur in a well-defined belt in the sedimentary rocks, following the stratification and approximately parallel, therefore, to the contact mentioned above. The stratification varies from N. 15° W., with a dip of 75° NE., in the vicinity of Rumichaca, to N. 50° W., with a dip of 60° SW., in the vicinity of Llacsacocha. (Cocha is the Indian (Quichua) name for “lake,” so Llacsacocha “lake” would be superfluous.) Asphaltite-veins have been found over a distance of 15 miles.
The veins occur as lenses, varying from 0.5 in. to 22 ft. wide, the maximum known length of a lens being about 500 ft. These veins are not necessarily confined to one bedding-plane, and may not only break through from one to another, but there may be asphaltite to the extent of 5 ft. in width, filling each of two or three contiguous bedding-planes, so that there appear to be three veins separated by single layers of shale from 8 to 10 in. thick. There is evidence of movement subsequent to the intrusion of the asphaltite, which is shown by the pencillate structure of the asphaltite, and numerous faults of small throw.
The asphaltite is black, lustrous, and softer than ordinary bituminous coal. The hardness does not appear to be affected by the amount of ash present. The material lacks completely any evidence of clay-bands and the columnar structure common to most bituminous coals. It breaks clean from the walls, though the country-rock adjoining often contains a large amount of carbonaceous matter. No pyrite was observed in the asphaltite at any of the exposures.
The mode of occurrence of the asphaltite is essentially the same at the various exposures marked on the map, so that only the most important, that on the north side of Llacsacocha, will be described.
The vein has been developed by five tunnels, aggregating about 2,000 ft., within a vertical elevation of 300 ft. The tunnels are from 150 to 500 ft. long. Table I., a record of samples from Tunnel No. 2, serves to explain in detail the variation in the width of the vein, and the amount of vanadium contained in the asphaltite
Table II. shows the amount of ash and of vanadic oxide contained in the samples from the various tunnels.
The series of proximate analyses in Table III. were made in the hope of recognizing some relation between the possible metamorphism of the asphaltite and the proximity of the eruptive contact, but such does not appear to be the case. Nor was it found that there was any apparent relation between physical properties and constitution.
It is apparent that the percentage of ash in the asphaltite from the Yauli district varies considerably. Further, the amount of vanadic oxide in the residue after burning varies between wide limits. This appeared to suggest that the vanadium was independent of the mineral matter in the asphaltite and that, therefore, its origin might be traced to the hydrocarbon portion.
The last column in Tables I., II., and III. gives a factor de-
rived by dividing the percentage of vanadic oxide in the asphaltite by the percentage of hydrocarbon, considering all combustible matter as hydrocarbon. It appears to me that this factor is constant enough to be considered an index of the solubility of vanadium (probably as the sulphide) in the hydro-carbon. This matter will be referred to later.
About 100 ft. east of the asphaltite-vein there is an outcrop of a dike of devitrified perlitic obsidian about 50 ft. wide. It appears to have been intruded along one of the bedding-planes. There are no contact-phenomena other than a noticeable hardening of the shales, though the dike appears to have been the cause of the formation of a zone of pyrite more or less replacing the shale in the footwall of the asphaltite-vein.
Quisque District Geology
Historical.—About Nov. 20, 1905, a party of Indians, who had been in the mountains searching for coal, brought to Senor AntenorRizo Patron, metallurgist at the Huaraucaca smelter, on the Cerro de Pasco railway, samples of a material thought
to be coal. The material came from a prospect near the crest of the main Cordillera, within the concession of the hacienda Quisque, the Indians having been led in this direction in the hope of finding an extension of the coal-fields of Oyon, 20 miles north. The prospect had been located for coal no less than three times previously, but was abandoned in each case when it was found that the material contained a large amount of sulphur. Under these old locations the prospect became known as Minasragra.
Upon analysis, the material was found to contain a high percentage of vanadium, which fact was subsequently confirmed in the laboratory of the Corps of Engineers in Lima. I was able to see the prospect in January, 1906, and as engineer for the American company which purchased the property, I have subsequently made two visits to the prospect.
Geology
The geology can best be explained by reference to Figs. 3, 4, 5, 6, and 7.
The area under consideration lies along the western limb of a broad anticlinal in Jura-Trias and Cretaceous rocks, similar in character to those exposed near Yauli, and over a large area along the axis of the mountains in this portion of Peru. The section shows the series in this locality to be composed of green shales, thin-bedded limestones, and red shales. The red shales are succeeded to the west by a great thickness of limestones, of which the main range is composed. The vanadium-deposit occurs entirely within the red shales.
Igneous activity in the form of the intrusion of dikes, laccoliths, and domes, has been a feature throughout a large area in this portion of the Province of Pasco, and these forms have been controlling factors in determining the local topography. It is evident from the map, Fig. 3, that this locality has been a unique center of this activity, there being no less than four systems of dikes, and the entire area being probably underlain by a laccolith.
The following rocks have been identified :
Dike B’.—A typical trachyte in an advanced state of decomposition. Contains red orthoclase, hornblende, and much secondary magnetite and calcite. Biotite and quartz are absent.
Laccolith E.—Dolerite, much altered. Contains oligoclase (?); short prisms of augite; idiomorphic olivine, slightly altered ; laths of hornblende; pyrite, calcite, original and secondary magnetite.
Dike C.—Typical andesite, comparatively fresh. Contains phenocrysts of oligoclase; laths of hornblende, and some biotite in a ground-mass of feldspar. This rock bears some resemblance to dikes A and D, though it shows more alteration.
Dikes A and D.—Typical quartz-porphyry, as shown in Fig. 13. Contains predominating plagioclase in phenocrysts; ortho- clase, generally corroded; quartz as rounded grains, and as comparatively perfect di-hexagonal pyramids; biotite, in a ground-mass of feldspar fragments. The feldspars from four
specimens are crushed, due to stress. There can be no doubt that dikes A and D are derived from the same magma, and probably are contemporaneous.
Dike B.—Diabase, without olivine, slightly decomposed. Contains decomposed hornblende; plagioclose; much magnetite, both original and secondary; no biotite, quartz, or olivine.
The following order of intrusion is deduced from the extra-ordinary number of intersections of the dikes, and the unique faulting: 1, dike B’; 2, laccolith E; 3, dike C; 4, dikes A and D (contemporaneous); 5, faulting: 6, dike B.
From the evidence of stress during solidification in dikes A and D, and the lack of it in dike C, it appears that the faulting began when A and D were yet semi-molten, but when C was completely solidified. Furthermore, A and D are fresh rocks, and C shows a considerable amount of decomposition, which could scarcely be attributed to atmospheric weathering.
A study of the map, Fig. 3, will show the faulting to have been of an extraordinary nature. Proceeding both eastward and westward from the crest of the ridge, where the laccolith outcrops, the faulting has been progressively southward. Further west from the vanadium-deposit the faulting is again reversed. It appears, therefore, that the area under consideration has
undergone faulting due to stresses resembling torsion in the earth’s crust.
Of the five intrusions, the laccolith is the only one which appears to have strictly followed the stratification of the rocks. Though dikes B and B’ have a strike corresponding to that of the shales, they are vertical, as shown in the section. Unusual contact-phenomena were not recognized in connection with any of the dikes, though the green shales overlying the laccolith are hardened.
The Vanadium Deposit
The vanadium-deposit proper is a lens-shaped mass composed principally of three distinct constituents, which occupies one of the faults of the dike A. The maximum width of this mass is about 28 ft., and though the length is partly concealed, it cannot exceed 350 ft. The strike is about N. 20° W., and the dip is 75° W.
The three materials in order of the relative amount present are :
Quisqueite; a black, lustrous hydrocarbon; hardness, 2.5; sp. gr., 1.75 ; fracture, conchoidal. Name derived from the hacienda in which the deposit is located.
Coke; a dull black, vesicular hydrocarbon; hardness, 4.5; sp. gr., 2.4; fracture, conchoidal. This coke contains globules of quisqueite.
Patronite; a greenish-black mineral; hardness, 2.5; sp. gr., 2.65 to 2.71; fracture, uneven. Name derived from that of Senor Antenor Rizo Patron, who first recognized that the mineral contained vanadium.
The following minerals, found in very small amounts, were recognized under the microscope :
Bravoite; a reddish-yellow mineral in patronite. Composition, (Fe, Ni) S2; hardness and specific gravity not known. Name given by Dr. Hillebrand in honor of Senor Jose J. Bravo, who has described the deposit.
An undetermined silicate mineral resembling halloysite.
Analyses of these minerals made by Dr. Hillebrand are :
For comment upon these analyses, and the action of various reagents on the materials, as throwing light on their constitution, see the original article by Dr. Hillebrand.
The specimens of patronite which were analyzed by Dr. Hillebrand were not as pure as some I have since been able to obtain. Analyses of pure material yield from 19.3 to 24.8 per cent, of vanadium, and the siliceous matter is lower than shown in the analyses by Dr. Hillebrand. I believe that the siliceous matter and possibly the carbon may be considered impurities, and that patronite approaches in composition a compound of vanadium and sulphur, which can best be represented by the formula V2S5 + nS.
The following extract from an article by Kay on The Sulphides of Vanadium has some bearing upon this phase of the question:
“ At a temperature of about 400°, vanadium trisulphide takes up two additional atoms of sulphur, forming the pentasulphide, V2S5. For the preparation of this compound the trisulphide is mixed with one-third its weight of sulphur (purified by carbon bisulphide), the mixture very finely powdered and placed in a strong narrow tube. The tube, after filling with dry carbon dioxide, is temporarily closed by a cork provided with a narrow capillary tube (to prevent access of oxygen), and the tube is then sealed off as near as possible to the surface of the mixture. The sealed tube is next heated in a hot-air oven for three hours, to a temperature of about 400°. The product, after washing with hot carbon disulphide to remove any free sulphur present, leaves a residue of the pure pentasulphide.
It is inadvisable to use a large excess of sulphur in the preparation of this sulphide, for although the pentasulphide is produced, it retains from one to two per cent, of sulphur very tenaciously, and the removal of this is a matter of considerable difficulty.”
I am unable to say whether the compositions of quisqueite and of the coke vary from the analyses given, for these are the only complete analyses that have been made. By analogy to many other natural hydrocarbons, I believe that the composition may vary within a small range from that given.
The nature of patronite and its relation chemically and genetically to quisqueite in coke will be made clear by a close study of the photomicrographs, Figs. 8 to 13. No photomicrographs have been made of the purest patronite, but an examination of several sections under a magnification of 200 diameters, after polishing and etching with caustic potash, showed a perfectly homogeneous surface, whose relief only varied with the degree of attack by the reagent.
The photomierographs show patronite, quisqueite, coke, bravoite, and the siliceous mineral, in varying proportions. In Figs. 8, 9, and 10, quisqueite is the black material, generally in the form of globules, though sometimes only a thin film lines the cavities, as in Fig. 10. The coke is black, but much duller in luster than quisqueite, and is found in the sections as an irregular net-work resembling a sponge. Patronite fills all of that portion not occupied by the coke and quisqueite, and is the light material showing the greatest relief in Fig. 10. Bravoite, which is of very irregular occurrence, is shown only in Fig. 12 as the unetched crystal in the darker ground-mass of patronite. The white segregations in Fig. 8 are the siliceous mineral referred to above.
Taken in connection with the larger structural relations shown in Fig. 7, it is clear that the materials are segregations from a mass which was probably originally homogeneous. Quisqueite was the first material to segregate. The larger portion was crowded to the walls, though a small amount was caught as globules in a viscous mass remaining. It probably segregated through insolubility in the remaining mass, rather than on account of any difference in melting-points, for it appears to have been viscous after the segregation of the coke. Moreover, it was probably unstable at the temperature then existing, this being suggested by the globules, which are more or less coked. The coke segregated after the quisqueite, and though nearly pure carbon, must have had the consistency of a paste, for it was able to form solid, fairly homogeneous masses.
Before the solidification of the patronite took place, there must have been a disturbance throughout the mass, due probably to its upward movement. This is shown by a crushed condition in the coke, Fig. 11, and to a less degree in the quisqueite, Fig. 9.
Patronite was the last mineral to solidify, and from its associations appears to have been the eutectic of the mass. It fills all of the cracks and spaces between the globules of quisqueite, Figs. 9, 10, and 11, and the walls of the cavities. The fact that patronite is of the nature of a eutectic serves to explain why its composition does not accord with the usual valency of vanadium, as eutectics, though generally constant in composition, are seldom compounds to which a formula may be assigned.
Patronite appears to have had the peculiar property, under the conditions of temperature and pressure which existed at the time of the intrusion of the mass, of being able to permeate the porous country-rock, even to the degree of saturating it. This condition is found in the hanging-wall of the mass, and the impregnation is generally in the neighborhood of a veinlet of quisqueite, suggesting that this latter material follows a fissure which was also the means of access of the patronite.
At some period, after the mass had become solid, a conclusive movement reopened the line of fault, rending the mass from end to end, as shown by the clay-filled fissure, Figs. 5 and 7.
The red shales are not only crushed, due to the faulting prior to the appearance of the mass, but much bleached, due probably to the action of the sulphurous vapors which accompanied the intrusion. The portions of dike A near the fault are also much decomposed, the feldspars being completely altered to kaolin. Explorations from the tunnel at a depth of about 50 ft. from the surface show the shales to be much silicified, and to be replaced by irregular zones of fine, granular pyrite.
Effects of Oxidation
The development at the time of the last inspection, August, 1907, consisted of an open-cut,, a tunnel about 270 ft. long, and six trenches, of which five cross the zone of intrusion, Figs. 3 and 5. This work facilitates a more thorough understanding of the nature and size of the deposit, and of the effects of oxidation, than was theretofore possible.
It is evident from the contours on the map, Fig. 3, that dike A determines the drainage of much of the area. Through the oxidation of the sulphide, patronite, there have been formed solutions of vanadium which, passing north and south along the west side of the mass, have precipitated oxide ores of vanadium. In some cases the solutions have almost entirely replaced portions of the shales, and at other places the vanadium minerals have been precipitated in the cracks and open spaces in the crushed zone. The mineralization of the shales has generally taken place along well-defined zones, though in places rich shoots of ore have been formed which pass into lean ore in the walls. Furthermore, where upon the surface there are found large areas of low-grade ore with occasional rich streaks, there are found at the tunnel-level (maximum vertical depth about 120 ft.) the same richer portions confined within barren material.
Two minerals of approximately definite composition have been found upon the surface. Both are hydrated oxides of vanadium, and though partial analyses only have been made, they appear to be new species. Dr. Hillebrand is inclined to regard them as salts, not as oxides or acids. The first, which will be called the “ red oxide,” has been analyzed by Dr. Hillebrand, with the result given below. It occurs typically as globular aggregates with radiated structure, though amorphous material is common. The color is deep brownish-red to red; streak, red; sp. gr., 2.30 to 2.48; hardness, 2.5. The second, which will be called the “ brown oxide,” has not been analyzed in detail. One analysis that I made shows: loss on ignition, 19.0; and vanadic oxide, 72.50 per cent.
The brown oxide is found along the outcrop in irregular amorphous masses, none having a tendency towards more definite structure. It is dark brown in color; streak, dark brown, sectile; hardness, 2.0 ; sp. gr., 2.30. It has the peculiar property of swelling and disintegrating when placed in water.
At a depth of 8 or 10 ft. from the surface, the red and brown minerals are replaced by a mineral having a greenish-black color, occurring under very much the same conditions. The mineral is generally amorphous, though along openings or water-courses it appears velvety, which appearance, under the microscope, is resolved into aggregates of acicular crystals, much resembling a common form of malachite. The specific gravity of the mineral is 2.52. Both analyses in Table V. were given by Dr. Hillebrand in a personal communication to me.
I suggested to him that the “ red oxide ” may be orthovanadic acid (H3VO4), which has not been known in nature heretofore, and that the “ green oxide ” may be a combination of V2O4 and V2O5 in approximately constant proportions. Analyses of three samples of this “green oxide” gave the following results:
Dr. Hillebrand has replied to these suggestions as follows:
“ You see that the proportion of V2O4, to V2O5 in the second of my analyses (green oxide) does not bear out your suggestion, when compared with your ratios, that it is a definite proportion. There is, indeed, no reason to expect other than a varying ratio between the V2O4, and V2O5 under the circumstances of occurrence. Every conceivable proportion might exist between the initial stage of oxidation of the patronite and the final stage represented by the red matter.
“ It strikes me that we must, from the nature of the occurrences, have mixtures of minerals in both cases. Until we know how the iron (especially the iron in the second analysis), molybdenum, V2O4, and lime are combined, it is useless to attempt to fix a formula for the V2O5 compounds, although quite permissible to indicate probabilities.
“ It appears that the ortho-, meta-, and pyro-vanadic acids are incapable of free existence. If formed momentarily, they pass at once into hexavanadic acid, H4V6O17, of which the orange incrustation (see below) seems to be a salt. The acid itself is, however, unstable, and in its further decomposition gives rise to separation of V2O5. I may add that the acid itself is not known in the free state, but its existence is assumed from the behavior of its solution in water. In view of the above, I am at present inclined to think that the red substance of which you furnished me specimens (red oxide), and which you think to be orthovanadic acid, may be a mixture of some calcium salt and of V2O5, possibly also of an iron salt. Against this, however, is the large amount of water shown by my analysis, an amount about equal to that found in the orange incrustation, but with apparently smaller amounts of bases. The presence of molybdenum in the material first analyzed, of which very little is present in the orange incrustation (see below), renders more difficult the forming of any conclusion regarding the nature of your supposed H3VO4. The same holds true for your H4V2O7, (green oxide), which, on account of the large amount of iron present in it, I am disposed to regard as essentially an iron vanadate. I am afraid that we had better not attempt to form any positive conclusions regarding the composition of these two products which are so evidently mixtures.”
It is probable that there is a gradual transition from vanadic oxide compounds on the surface to those of hypovanadic and vanadic oxides below the surface, though the large amount of iron shown in Dr. Hillebrand’s analysis makes his suggestion that the material which he analyzed was iron vanadate undoubtedly correct.
Solution of vanadium compounds is in process continually, and waters flowing from the tunnel show a considerable amount of vanadium in solution. The waters, percolating through the walls of the tunnel, deposit an orange incrustation, after analysis of which Dr. Hillebrand comments as follows:
“ I have finished an approximate analysis of the crystalline orange incrustation on one specimen which you sent me quite a while ago. This orange incrustation is apparently a hydrous calcium salt of hexavanadic acid, that is, Ca2V6O17 + x H2O. The x is between 10 and 11. Water is about 22 per cent., of which 14 per cent, comes off over sulphuric acid, if the exposure is prolonged for several months, after which time the exposure at 100° C. gives rise to no further loss. The mineral then loses little water until the temperature has gone up quite a bit, but at or below 250° becomes wholly dehydrated.
https://www.youtube.com/watch?v=j5Rs2ywfSzA
“The percentage of calcium oxide is about 12.8 percent., that of V2O5 about 65.4 per cent. There is also a very small amount of insoluble matter that has been deducted in deriving these figures.”
Table VI. is a record of analyses of samples prepared by quartering one wheel-barrow load, from trench B and from the open-cut on the north side of the dike, and gives an idea of the extent of the replacement of the shales by vanadium compounds :
The tunnel cuts this same zone of replacement at a depth of 120 ft. along the clip of the shales. The following samples show the diminution in the percentage of vanadium.
This impregnation, or replacement of the shales, can, of course, only extend to the depth of ground-water circulation, which in this immediate vicinity will probably not exceed 100 ft. vertically (about 200 ft. on the dip of the shales).
Production.—Within the period from June, 1906, to January, 1909, there has been produced and shipped to the United States about 1,800 tons of oxidized ores, containing about 20 per cent, of vanadic oxide; also the product obtained from roasting about 400 tons of sulphide ore, patronite.
Conclusions
The occurrence of vanadium in hydrocarbons is not confined to Peru, for it has been announced by Kyle in the ash from a coal (asphaltite ?) found in the province of Mendoza, Argentine Republic; also from other localities mentioned in Clarke’s Data of Geochemistry. I have found it in the ash from an asphaltite from Page, Indian Territory (now Oklahoma). The material contained 1.10 per cent, of ash, of which 0.19 per cent, was vanadic oxide. A review of the original articles describing the above mentioned occurrences has convinced me that all of the materials are very closely related, if not almost identical, in nature and occurrence. In other words, they all appear to be asphaltites containing an appreciable amount of sulphur.
Investigators of the subject seem agreed with the opinion expressed by Eldridge in his article, entitled The Formation of Asphalt Veins, that asphaltites are derived from petroleum. Richardson, after a most thorough study of the subject from a chemical standpoint, shows that all asphalts contain sulphur, and that the hardness appears to depend upon the amount present.
“ Asphalts are distinguished by the large amount of sulphur they contain, and it is to its presence that many of the important characteristics, and perhaps in part, the origin of this form of bitumen is due
“ The harder and least soluble portion always contains the larger part of the sulphur. It seems, therefore, that sulphur is the effectual hardening agent of natural asphalts, in the same way that it is of artificial asphalts which are produced by heating a soft natural bitumen with sulphur
“It seems justifiable, therefore, to suggest that where certain mineral oils, composed of alicyclic hydrocarbons, originate under such circumstances as to be subjected to conditions favorable to condensation and polymerization, or to the action of sulphur or sulphates, asphalt will be formed, not necessarily immediately, but in the course of time.”
Further, a method of preparing the sulphides of vanadium, described by Carnot, has some bearing:
V2S3 is prepared by heating an oxide or a chloride of vanadium in hydrogen sulphide, or vapor of sulphide of carbon. This sulphide, heated in a current of hydrogen, furnished the bisulphide. V2S2, and in the vapor of sulphide of carbon, at 400°, the pentasulphide.
Taking these observations into consideration, the occurrence of vanadium in asphaltites appears to depend upon three factors:
- Vanadium, as oxide, disseminated through a rock of a fair degree of porosity.
- Impregnation with a hydrocarbon to a greater or less degree.
- A source of sulphur or sulphureted vapors.
The first of these conditions undoubtedly often exists over large areas. In the two districts in Peru under consideration, the two remaining conditions have undoubtedly been brought about by the intrusion of the dikes. It would seem, therefore, a safe forecast, that most asphaltites containing more than 2 per cent, of sulphur contain vanadium.
The Quisque deposit may now be interpreted as an extreme phase of differentiation from asphaltite, the intrusion of the dikes probably having had the effect of successively concentrating the vanadium. Further, the unique climatic conditions account fully for the formation of the large aureole of oxidized ores. Had there been erosion by water even to a slight degree, the oxidized minerals would have been carried away, instead of being permitted to accumulate in the porous country-rock.
With the exception of the analyses made by Dr. W. F. Hillebrand, of Washington, to determine the composition of the minerals, and those in Table VI., which were made by J. O. Handy, of Pittsburg, Pa., I have done all the analytical and research-work given in the present paper. Cordial acknowledgment is hereby made to Dr. Hillebrand, whose analytical work has been of invaluable assistance.
Acknowledgment is also made to Senor Felipe de Lucio, mining engineer in charge of development of the property, for unqualified co-operation in every phase of the investigation and work on the property.