Table of Contents
X-ray fluorescence or XRF is an analytical technique that has been used for many years to determine the elemental composition of a wide variety of materials. Examples include metal alloys, minerals and petroleum products. X-rays form part of the electromagnetic spectrum. They are on the high energy side of the ultraviolet and are expressed in terms of their energy in kiloelectronvolts or wavelength in nanometers. XRF can typically analyze elements from sodium to uranium in concentrations ranging from parts per million to high percent in solids, liquids and powders. Of course, the elements and concentrations that XRF analyzers can determine depend on the material being tested and the instrument used.
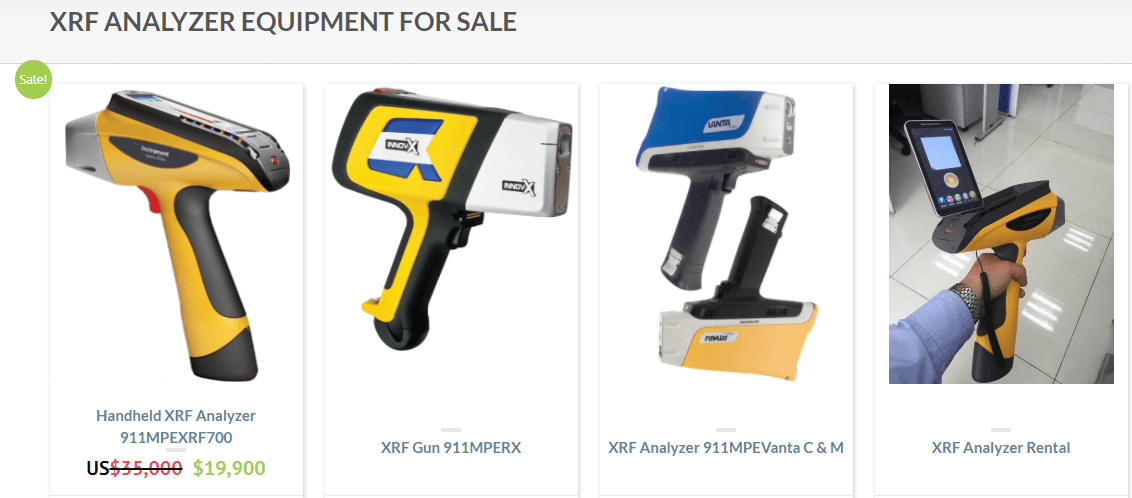
So, how does X-ray fluorescence work? All XRF instruments are designed around two major components: an X-ray source, commonly an X-ray tube and a detector. Primary X-rays are generated by the sources and directed at the sample’s surface, sometimes passing through a filter to modify the X-ray beam. When the beam hits the sample, they react by generating secondary X-rays that are collected and processed by a detector.
Now, let’s look at what happens to the atoms in the sample during the analysis. A stable atom is made out of a nucleus and electrons orbiting it. The electrons are arranged in energy levels or shells, and different energy levels can hold different numbers of electrons. When the high energy primary X-ray collides with an atom, it disturbs its equilibrium. An electron is ejected from a low-energy level and a vacancy is created, making the atom instable. To restore stability, an electron from a higher energy level falls into this vacancy. The excess energy released as the electron moves between the two levels is emitted in the form of a secondary X-ray. The energy of the emitted X-ray is characteristic of the element. This means that XRF provides qualitative information about the sample measured, however XRF is also a quantitative technique.
The X-rays emitted by the atoms in the sample are collected by a detector and processed in the analyzer to generate a spectrum showing the X-ray’s intensity peaks versus their energy. As we have seen, the peak energy identifies the element. Its peak area or intensity gives an indication of its amount in the sample. The analyzer then uses this information to calculate the sample’s elemental composition. The whole process from pressing a start button or a trigger to getting the analysis results can be as quick as two seconds, or can take several minutes. Compared to other analytical techniques, XRF has many advantages. It’s fast. It measures a wide range of elements and concentrations in many different types of materials. It’s non-destructive and requires low or very little sample preparation. And it’s very low-cost compared to other techniques. That’s why so many people all around the world are using XRF on a daily basis to analyze materials.
Fundamental XRF Principle of Operation
So now I want to take a minute to talk about how XRF is able to provide these real-time quantitative values. Using an X-ray tube, we start by creating and directing an X-ray beam into the sample material. The lighting up of the sample occurs when the X-ray energy temporarily knocks and electron out of its orbital, ionizing an atom. In the process of transitioning from a positive ion back to electrically neutral, the lid up sample emits an X-ray energy that is specific for each element. The detector captures the spectrum of energy is displayed here and beyond calculating the chemistry from the spectrum, the elemental values can be used to determine the grade match.
What’s happening in the algorithm basically is we’re correcting for background interferences from other elements. Once we get a net signal for each element we’re able to apply our calibration algorithm and determine the percent concentration. This process as far as the user is concerned is really real time. The test starts and the answer is directly displayed.
The emitted energy in real time is turned into an elemental analysis as you can see on the right of the slide. The algorithms are different from application to application, but all of them turn in a quantitative result. While the slide diagrams we’ve been looking at, show single atoms, in reality tens of thousands of signature X-rays are captured by the detector every second. As long as the sample’s surface is representative of the entire sample, fast, accurate and quantitative results can be achieved in just a few seconds.
This slide is meant to bring your attention to the fact that field X-ray analysis for analytical purposes is a near surface test. In the diagram we have something that is meant to be the thickness of a human hair called a piece of stainless steel that’s 70 microns in diameter. If analyzing that with the Fox IQ system the depth of penetration would be only 10 microns. So the reading depends on the surface being representative of the entire sample.
Elemental limits run from phosphorous to plutonium; typically based on customer needs analyzers are set up to measure from titanium to bismuth. By the end of the calendar year, the elemental range would be extended to aluminum as an alloy in material, iron, copper or titanium grades. Typical applications.
How X Ray Fluorescence Work
The analyzers operate using energy-dispersing X-ray fluorescence EDXRF analysis. Samples are excited by high energy photons produced by the XL3 source. Sample chemistries are quickly, non-destructively determined as the characteristic fluorescent X-rays for each element plus Compton and Rayleigh scatter from the sample are measured simultaneously by the X-ray detection system.
Let’s begin by taking a look at electrons and how they generate energy. Every atom contains a positively charged nucleus and one or more electrons in quantum energy states, or orbital shells surrounding the nucleus. For elements heavier than neon that contain 3 or more orbital shells, the three lowest potential energy orbital shells are the K shell, L shell and M shell. When a sample is radiated by sufficiently high energy photons generated by either a miniature X-ray tube or a sealed radioisotope source in the analyzer, electrons from the inner orbital shells of samples atoms are rejected from their orbital shells.
The atom instantaneously fills the electron vacancy as one of the electrons from a higher energy orbital shell drops to the lower energy state of the ejected electron, releasing energy in the form of a characteristic fluorescent X-ray. This move of an electron from a higher to a lower energy state creates the phenomenon known as X-ray fluorescence. For example, when the X-ray beam strikes the atom an electron from the K orbital shell is ejected. An electron from the L orbital shell drops to the K orbital shell. At the same time, this electron releases energy to move to a lower energy state. The fluorescent energy released then travels back through the XL3’s Capton window and into the instrument’s high-resolution X-ray detector.
Here you see the reading number which identifies the sample reading and the test mode used for the measurement. The reading number for this stainless-steel sample is 164 and we test it in the alloy mode. The measurement time for the sample measurement. For this sample, we ran it for a full 30 seconds. The battery strength: we can see the analyzer’s battery is at full strength. The alloy grade identification. This sample is a 316 stainless-steel. And the list of elements in the sample.
How XRF Works + Working Principle
The process of XRF has got 5 steps as we described it here. This is showing the window that I described on the front of the system that’s about the size of a dime. You would want that window to be on or near the sample. It doesn’t have to be exactly on it, but the sample has to be in front of the window. It’s best to put it on it. You pull the trigger to activate the X-ray tube and then steps 2, 3 and 4 are really things that the user doesn’t see. The X-rays excite the sample, knock an electron off and as part of that instability, the system shifts its electrons around, emits a photon of energy that is characteristic to the element. We capture it as part of a spectrum of energy and the part again that is visible to the user is that we come up with a great match and a measured chemistry on the display. So pull the trigger, see the result; these steps are the things that are going on in-between.
Here’s another view of the same information. We’ve got a Bohr model of the atom nucleus; electrons, orbital that the electrons are in. We shoot in our X-ray, an electron is knocked out of its orbital, knocked out of its shell. The result is that the electrons shift to go back to the electrically neutral and there’s a fluorescent photon that goes out and that energy will be specific to the atom that we’ve excited. So every element has a different signature energy that it emits.
And this is just another, an animation of that process. Now, of course we show ourselves ionizing four atoms and getting 4 different photons back, but in reality XRF is a statistical method and we can be exciting up to 100,000 photons in a second, not 4 in 4 seconds. And we process that information to determine the concentration in the sample. Here you’re looking at the spectrum and chrome has a lower energy than iron, than nickel, than moly and the height of the peaks is directly related to the concentration of the elements. So, if there were twice as much chromium, we would have twice as high a chromium peak and so forth. And then the part that is visible to the user is the display where the chrome comes up, and that is a peak in the energy spectrum as the amount of chrome in the sample.
So, the way that it works is that we catch the spectra, we subtract out interferences, we make corrections for background and interfering elements. We apply our calibration factors to the net signal and then what comes out the other end is the elemental concentrations. The elements that we can test are circled here by the red boundary, basically from magnesium to plutonium is what we can do. Magnesium is the lightest element that we can test. The things that we can’t do that are common to real-world applications and to allow testing would be things like carbon, oxygen, nitrogen, sometimes beryllium or boron. Anything lighter than magnesium, a lower Z number in this chart would not be measurable. We measure these elements, we normalize to 100% – it allows us to correct for size and shape and that’s the method that’s used.
Today we got in a couple pieces of angle. When you look at the angle it looks so similar that we’re not always sure what it is. So, when we use our analyzer, we make sure to go up to it, have a nice clean surface, pull the button and right now this is telling us that this is an aluminum 6061.