Table of Contents
The electric furnace has always been found to be especially adapted to melting, refining, and finishing processes throughout its gradual acceptance by metallurgists as a practical apparatus for conducting metallurgical operations. In the steel industry, the electric furnace is firmly established in the manufacture of steel of the highest grade, equal to crucible steel. For the production of the cheaper grades of steel in large tonnage, the electric furnace gives a higher-grade product than the open-hearth or converter, but due to the high cost of power prevailing in steel centers, considered from the electric furnace standpoint, it is not economical to produce tonnage steel in the electric furnace. As a refining and finishing agent for open-hearth or Bessemer steel, the electric furnace has had some degree of success in the production of tonnage steel.
After a study of the use of the electric furnace in the steel industry, it appears that there may be a possibility of its use to advantage for the melting of cathode copper. In the case of copper the problem is not one of actual refining, because the copper if refined properly by the electrolytic method needs no further refining; it needs simply to be cast into a marketable shape. The name “refining” is applied to the present finishing process, because in the operation oxygen and other impurities are removed, which the electrolytic copper absorbs in being melted in the reverberatory furnace. Considering that processes may be positive, negative, and neutral, the electrolytic method would be positive; reverberatory melting of cathode copper, negative; and electric furnace melting, neutral. The electrolytic method produces about as pure a copper as is possible, if operated properly and the anodes are not too impure. The final reverberatory melting of this cathode copper lowers the grade of the final product, due to the absorption of gases and impurities.
The reverberatory melting of cathode copper is one of the weak parts of the metallurgy of copper to-day. A refining process is not required, for before the copper is melted in the reverberatory furnace it is pure. The ideal process would be one in which the cathode copper could be melted and cast into marketable shapes without absorbing impurities; i.e., a neutral process.
Reverberatory Furnace Refining
As cathode copper is practically pure copper, it would be ready for use if in a marketable shape, but as it is not, the cathodes must be melted. Common practice is to melt cathodes in the reverberatory furnace. The practice at Great Falls, Mont., has been described by W. T. Burns as follows:
Two coal-fired reverberatory furnaces of 100,000 lb. capacity each per charge are used for melting cathodes. The coal used in firing them contains about 3.5 per cent, sulphur. On account of this high sulphur content in the coal, it is necessary to protect the copper as it melts from the sulphur dioxide gases resulting from combustion of the fuel. In order to do so about 30 per cent, of the cathodes going to make up a wire-bar charge are dipped in milk of lime before charging. As the copper melts the lime forms a protective coating for the metal, thus hindering in a large measure the absorption of sulphur by the molten copper. Just before rabbling (or oxidizing) is started the slag is skimmed from the furnace. The slag obtained is equivalent in weight to about 3.5 per cent, of the copper charged, varying with the amount of lime added. The slag contains about 60 per cent, copper.
After the charge is completely melted, the usual practice is followed of rabbling and poling the copper for oxidation of the impurities and bringing the metal to the proper pitch for casting. Rabbling is effected by introducing compressed air at a pressure of 16 lb. per square inch into tho molten bath. The poling and reducing action is obtained by forcing green pine poles into the bath and by covering the surface of the bath with charcoal.
The condition of the bath, as regards oxygen content, is noted from time to time by the refiner as he examines the successive button samples taken from the bath in a small ladle. When the physical appearance of the button indicates that the “tough pitch” stage has been reached, the poles are removed and the dipping operation is begun.
Why Reverberatory Refining is Superfluous
The process of reverberatory melting of cathode copper as above outlined is not a refining process. That it is so called is due to the fact that it is necessary to remove the oxygen and other impurities which the electrolytic copper absorbs during melting in the reverberatory furnace.
That the furnace refining of copper is superfluous in so far as it improves the grade of marketable copper has been shown by the experiments of E. Keller.
As a result of these experiments Keller states that “electrolytic copper which has not been melted and refined is superior in conductivity to the refined and cast copper by over 2 per cent. There is, therefore, a field for improvement in the refining or making of wire from electrolytic copper without previous melting.” Peters states that “ordinary electrically deposited copper produced on a large scale at a plant using the Emerson system of depositing sheets direct without subsequent furnace refining showed a conductivity of from 102 to 103 per cent.”
Another test showing the furnace-refining operation to be superfluous, or even harmful, was made by rolling wire from a mass of Lake Superior native copper and, after annealing it, comparing it with cathode copper and furnace-refined native copper. The mass copper and cathode copper each had a conductivity of 102.5 per cent., while furnace-refined native copper had a conductivity of 99.5 to 100 per cent.
Effect of Cuprous Oxide on Copper
As before stated, in the reverberatory process, while the copper is being melted, it absorbs oxygen, sulphur and other impurities from the gas passing over it from the fire box of the furnace; when air is blown into the molten copper, about 5 to 6 per cent, cuprous oxide is found in the charge. This cuprous oxide gives up its oxygen to the metallic impurities in the copper, forming oxides, which pass into the slag. After the removal of all the sulphur dioxide gas the cuprous oxide is reduced to metal by poling until the button sample shows the proper structure and the copper is tough pitch, when it is cast. The cathode copper contains less than 0.1 per cent, cuprous oxide. After furnace refining it contains 0.4 to 1.2 per cent, cuprous oxide, with an average of about 0.7 per cent.
It seems that under the present conditions of refining, cuprous oxide is essential to the quality of the copper produced, as is shown by the fact that if the poling is continued below certain limits the quality of the metal becomes impaired.
It does not appear, however, that the presence of cuprous oxide itself has the direct effect of improving the quality of the metal, as mass Lake copper without previous melting shows a higher all around standard than furnace-refined Lake copper. Hampe found that the addition of cuprous oxide produced no perceptible effect upon the strength or malleability of pure copper until 0.45 per cent, was added, when a slight diminution of tenacity was observable. With increasing proportions of cuprous oxide, the quality of the copper suffered more perceptibly.
Addicks found that the addition of 0.05 per cent, oxygen (0.44 per cent. Cu2O) to pure copper increases slightly its conductivity, which drops back again to about normal when the addition of oxygen reaches 0. 1 per cent. (0.9 per cent. Cu2O) and decreases considerably by further addition.
The theory has been advanced that the presence of cuprous oxide improves the quality of the copper by preventing the reduction of the dissolved foreign oxides, harmless while oxidized but injurious when reduced to the metallic state. These impurities may also be reduced during over-poling to reduce the cuprous oxide.
Peters states, “We may, I think, say with safety that, while the proportion of cuprous oxide found in ordinary good refined copper does not appear to diminish its electrical conductivity (it may even increase it slightly), the very highest conductivity tests are yielded by the copper which contains no determinable oxygen and that cuprous oxide, in the proportion usually found in good refined copper, appears to have but little effect, one way or the other, upon the malleability, ductility, or electrical conductivity of the metal.”
Electric Furnace Melting of Cathode Copper
From the preceding discussion of present practice in melting cathode copper, it can be seen that the ideal process for melting cathodes is a method by which it is possible to produce cheaply in marketable form as pure a copper as is charged into the melting furnace. Such a process to be successful both metallurgically and commercially should conform to the following conditions:
- In order to keep the percentage of impurities as low in the final product as in the cathode copper charged into the furnace, there should be a neutral atmosphere in the melting chamber with a minimum possibility of introduction of air, gases, or impurities which might contaminate the copper.
https://www.911metallurgist.com/equipment/smelting/electric/ - Melting should be performed without excessive losses of copper, either by volatilization or in any slag which cannot be returned to the blast furnace or reverberatory furnace for re-smelting.
- The process should be susceptible to ready mechanical manipulation for charging and tapping.
- The cost of production should be at least as low as by existing methods, which means that the furnace must handle a large quantity of copper per day with an efficient use of labor, fuel, and power.
The reverberatory process possesses all of these qualifications with the exception of the first, for, as has been shown, air, gases, and impurities are absorbed by the copper and must be removed as far as possible later in the operation. It is not simply a neutral melting furnace. It melts copper cheaply, is easily charged and tapped, and has no apparent loss by volatilization, but produces a poorer grade of copper than it receives.
Factors Governing Use of the Electric Furnace
The use of the electric furnace for melting cathode copper would depend largely upon the cost of operation and the loss of copper by volatilization. The cost of operation would be influenced chiefly by the cost of hydro-electric power in comparison with the cost of coal, with the higher efficiency of the electric furnace in its favor. A loss by volatilization has been found to occur by experimenters using the direct-arc type of furnace with the copper covered with slag. There has been no loss in this manner with the indirect-arc type of furnace, heating by radiation of the arc, or in the resistance furnace.
This is a question requiring further experimenting. A large electric furnace is easily manipulated both for charging or discharging, as has been found to be the case with electric steel furnaces, and lends itself readily to the use of mechanical devices for charging cathodes and casting the copper.
Electric Furnace Advantages
Disregarding the commercial economy of an electric furnace for melting copper, the electric furnace provides an absolutely neutral melting chamber with no possible introduction of air or impure gases of combustion during the melting of the copper. Hence, since electrolytically deposited copper is practically pure, the copper melted in the electric furnace should be as pure as cathode copper, and subsequent oxidation and poling are not necessary to remove impurities acquired in melting, as none are acquired. That a product entirely free from oxygen can be produced by melting copper in the electric furnace has been shown, as stated in a previous paper by the writers.
Native copper concentrates which contained about 35 per cent, copper were melted in an arc furnace, the slag being formed by the gangue of the concentrate with a suitable flux. As a result of 17 tappings of the furnace, copper was produced in which no trace of oxygen could be found after etching with picric acid and studying the results with the microscope. To confirm these results, the same samples were submitted to E. S. Bardwell, of Great Falls, Mont., for etching with hydrogen and determination of oxygen by his method. After an examination of 12 samples Mr. Bardwell confirms the results of the writers by reporting that he can discover no oxygen in the samples after etching with hydrogen.
Types of Electric Furnaces for Melting of Cathode Copper
There are four types of furnaces which might be used for the melting of cathode copper:
- the induction furnace;
- the resistance furnace;
- the direct-arc furnace; and
- the indirect-arc furnace.
Of these furnaces the induction furnace is not practical, for the following reasons:
- The resistivity of the copper is so low that the current necessary to produce the required heat must be so great that the pinch effect severs the column of molten metal;
- the induction furnace is not adapted to melting cold metal; and
- the size and shape of the hearth would make it difficult to charge large quantities of cathodes. It is stated by one authority that when copper is melted in the induction furnace, the column breaks off so often that it is necessary to have a crucible of molten metal ready to pour into the break.
The crucible resistance furnace with a solid resistor is too small for melting large quantities of copper, and as now constructed is not practical in large sizes. A resistance furnace of the type used by FitzGerald, with a conducting roof which acts as a resistor and from which heat is reflected to the metal beneath, would not be practical in very large sizes because of the weakness of the roof. Also this type of furnace was found to have a low thermal efficiency.
Electric furnaces of the direct resistance type in which the molten bath is the resistor have not been successful in the steel industry, and attempts to use this principle have been abandoned. The recent use of the “pinch phenomenon” in resistance furnaces with a molten resistor, however, has been successful in small installations. Even in melting steel in such a furnace it is necessary to use a very low voltage to get the high amperes necessary for heating. The transformers are attached to the bottom of the furnace, which, while all right in small sizes, might cause complications in a large furnace. With copper in the furnace an even lower voltage would be necessary, adding to the difficulties of design. For molten steel a voltage of from 6 to 10 volts has been necessary.
The pinch furnace does not seem adapted to a large capacity per charge, and as yet has not been built in sizes approaching arc steel furnaces. While it might be used for melting about 500 lb. of cathode copper per charge, with short successive runs, this scheme does not seem practical for a plant melting 100 tons of copper per day. When copper was tapped at intervals of about 10 min., it would be difficult to use large-scale charging and casting machinery, which reduces the labor cost very much. The wear on the lining of the furnace would be greater than when a large furnace is used and discharged at intervals of several hours.
Another disadvantage of such a furnace is that in reverberatory melting large amounts of copper are absorbed by the lining, and this would doubtless be the case also in electric-furnace melting. This would prove a great disadvantage to the use of an electric furnace with a conducting hearth of any sort. While the hearth of the pinch furnace is not conducting over its entire cross-section, it is conducting at the points where the injectors or electrodes are placed in the hearth. After absorption of copper by the hearth short circuits might occur between the electrodes, and if the furnace were relined before affairs could reach this point, it would be a case of relining the furnace very often, at great expense. In the case of a furnace with a non-conducting hearth, the copper could be permitted to accumulate on the hearth without injuring the operation of the furnace.
In regard to the use of the pinch furnace for melting cathode copper, there is no question that it gives the best metallurgical conditions for carrying on the operation in a neutral atmosphere, but, in the opinion of the writers, it does not seem, from present developments, to be certain of success for this purpose in a practical way on a large scale. There are too many problems to be worked out in regard to the practical application of this type of furnace to warrant its use for tonnage melting at the present time. It provides a neutral atmosphere, with no chance of the introduction of impurities, and has one advantage over the direct-arc type of furnace in that there is not the necessity of keeping a bath of slag over the copper to prevent loss by volatilization. It is practically impossible for any volatilization of copper to occur in a pinch furnace.
Another possible difficulty in the use of the pinch furnace on a large scale is the necessity of keeping a molten bath of copper in the furnace in order to operate it. In charging, cold solid copper would be placed into hot molten copper. If there was any splashing of the copper, there might be a tendency for copper to collect on the roof, as the roof is the cold part of the furnace, since all the heat is generated in the metal charged.
The indirect-arc furnace in which an arc is drawn between electrodes, and in which the passage of the current is entirely independent of the molten bath, has the advantage of having the heating independent of the slag or metal. In steel melting the very basic slag used tends to promote the steadiness of the arc. Hansen states that the arc in a zinc furnace or brass-melting furnace of this type is rather snappy and unsteady. This is apparently not fatal, for in his experiments with the Weeks furnace the arc was operated for 42 hr. continuously in an atmosphere of zinc vapor. The electrodes were not adjusted once during this time.
For copper melting on a large scale a furnace with from three to six electrodes probably would be used. Under such circumstances it would be necessary to have the electrodes controlled hydraulically by hand, as in the indirect-arc steel furnace, or by automatic electric control. From experience with the indirect-arc steel furnace it is evident that with furnaces of this type difficulty occurs in the regulation of electrodes and the regulation of the arc for a capacity of over 2 tons per charge. The larger size seems to present complications too great to permit of satisfactory operation. The 5-ton furnace built at Turin failed largely for this reason, and in steel melting it has been evident that the indirect-arc furnace is not adaptable in sizes of over 2 tons, or 300 kw. power load. In his first trials with the electric furnace, Stassano built a rectangular hearth furnace with six horizontal electrodes arranged in three pairs, lengthwise of the furnace. This furnace failed largely because of the difficulty in maintaining the arc and the lining.
One of the greatest objections to the indirect-arc furnace for steel manufacture has been the high repair cost for linings resulting from the direct radiation of the heat of the arc. Magnesite linings, which increase the expense considerably, are necessary. The heat losses in water-cooling the electrodes are also larger than in some other types of electric steel furnaces.
As a result of experience with the indirect-arc steel furnace, while there would be less chance of loss of copper by volatilization than in the direct-arc type, it is believed that the furnace would be too complicated for practical work; the up-keep cost too expensive; and the heat losses high. This furnace, while giving the proper atmosphere for carrying on the melting of copper, does not seem to be a practical, efficient furnace for large-scale work.
The direct-arc furnace, in which the arc is formed directly between the electrode and the slag or metal, may be of the conducting or non-conducting hearth type. The conducting hearth type is not feasible for copper melting, as before stated, because it has been found that the combustion reverberatory furnace used in copper melting absorbs large quantities of metallic copper. Also there is always the danger of metal breaking through the bottom of a conducting hearth, which would be more apt to occur in the case of copper than steel, on account of the lower melting point and greater fluidity of the metal.
After a careful study of the existing types of electric furnaces, it is believed that the most practical furnace for copper melting would be the direct-arc type, having a non-conducting hearth, as for example the Heroult steel furnace. As an efficient, practical furnace, this furnace has demonstrated its superiority in the metallurgy of steel. The objections to the direct-arc type as not affording theoretically the best possible conditions for copper melting do not, in the opinion of the writers, overweigh the practical points in its favor.
There are three chief disadvantages to the direct-arc electric furnace for the melting of cathode copper:
- In order to prevent volatilization it is necessary to keep slag on the bath at all times when current is passing through the furnace.
- From experimental work to date, it appears that there is a slight volatilization of copper with a furnace of this typo even when a slag is used.
- The presence of slag makes it difficult to discharge the furnace and keep the copper at the proper temperature for pouring.
With the exception of loss by volatilization, these objections do not seem serious, and the volatilization loss should be very small if the furnace is operated largely as assistance furnace with a thick enough bed of slag (2 to 3 in.) to prevent direct arcing with the metal, and a low current density in the electrode to reduce excessive local heating at the points where the electrodes dip into the slag. Hansen states that in a newly lined furnace not designed for copper melting he tapped 99 per cent, of the metal charged. The actual loss could be determined only after a campaign of several months. If loss occurred, it would probably be at the start of a run when the slag is cold and not fused.
The question of discharging the furnace is a problem of operation. The copper could be cast by hand from a ladle, as is done at Great Falls. In this case it would be necessary to preheat the ladle and skim the slag from it before pouring, if the slot tap hole usually used on reverberatory melting furnaces is employed. Or a preheated bottom-casting ladle could be used, as in making steel castings. A siphon tap hole might be designed so that the copper drawn came from the bottom of the bath, leaving the slag on top of the metal. This tap hole would be lowered gradually, as in present melting practice, taking the metal from the bottom, but with only the pressure of the surface of the bath behind it. Only the last of the copper drawn would contain slag, and the current could be maintained on the furnace enough to keep the copper at the proper casting temperature until the slag was lowered to the bottom of the furnace. Thus bottom casting, if preferable to skimming in the ladle, would not be necessary except at the end of the operation.
For machine casting, with a siphon tap hole the operation would be performed as in reverberatory practice, except that it would be necessary to skim the last ladles or use a bottom-casting ladle on account of the slag. It is simply a question of manipulation.
In the electric process of melting cathodes with the direct-arc furnace, the charge would be placed in the furnace mechanically with a charging machine, as in reverberatory practice. The electrodes would be drawn up during charging. After the cathodes were all added to the furnace, lime and silica would be shoveled in to form a protective covering of slag. The slag should be of as low a melting point as possible. The electrodes would then be lowered, the furnace shut up and the circuit closed. The electrodes would be regulated by hand at first and later by Thury regulators, as in steel furnaces. Under these circumstances, as the slag is practically pure and no air is admitted into the furnace, poling is not necessary and the furnace can be tapped when the charge is all melted and at the proper casting temperature. During tapping the electrodes would be gradually lowered and the current kept on, so as to maintain the proper temperature. When operated with a slag 2 to 3 in. thick, the furnace is really a resistance furnace rather than a direct-arc furnace.
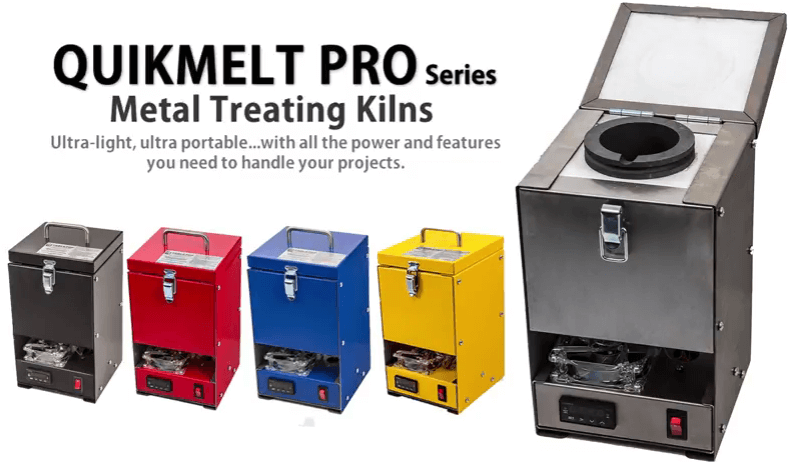
Because of the small amount of work done with melting of copper in the electric furnace, accurate estimates of the cost of the process are difficult to obtain. It is probable that the power consumption would not be over 300 kw-hr. per ton of copper melted. Using this figure and basing other expenses on electric-furnace steel practice, the cost of melting cathode copper in a furnace of 25 tons capacity per charge should not exceed $4.75 per ton, or 0.238c. per-pound, of copper melted, with power at ½c. per kilowatt-hour.
Conclusion
This short discussion of the subject is not offered with the idea of attempting to convert those interested in the matter to the belief that the only thing to do is to melt their copper in an electric furnace, but rather to suggest that the electric furnace might be used for that purpose.
We also hope that the paper may be the means of starting a discussion of the subject, by melters of copper and electric furnace men alike, for, as is well known, quite often it is not the paper itself, but the discussion of it, that makes it valuable, and so we hope it will be in this case.
Discussion
Lawrence Addicks, Chrome, N. J.—I read this paper for the first time this afternoon, and it is really worthy of a much more careful reply than I can make with such a short time to consider it, but I just want to say that I differ with Dr. Lyon in his premises and conception of what happens in a refining furnace. He cites steel as an instance where the production of special grades justifies the high cost of power in an electric furnace, and goes on to say the same will be true in the case of copper, for the reason that you will get a better quality of product. I don’t think you will get a higher grade of copper, or a better conductivity, as I will explain in a moment. The cost of fuel in the Eastern plants is somewhere between 30c. and 40c. per ton of copper refined, and, if my memory serves me correctly, in an open-hearth steel furnace it is something like $1.25, so it is evident that steel could be handled to better advantage than copper on the basis of fuel. Now, taking the Great Falls plant to compare with is not quite fair, because that plant operates under peculiar conditions. The furnaces are relatively small; the coal is very bad and is very high in sulphur. No one would think of using a coal with 3.6 per cent, sulphur were anything better available. Due to these conditions, the amount of slag is inordinate, it being quoted as 3.5 per cent., and it is necessary to use lime, as there is a tendency to have troubles from arsenic owing to the very high current density used. I think he has taken about as hard a case as he could pick out. Now as to the conductivity of copper, my own idea about the reason that melted copper does not give as high a conductivity as pieces drawn directly from a cathode, or native copper, is that in the latter case the impurities are present as a mechanical mixture, while after melting they are chemically combined with the copper, which is a very different condition. Further, it is necessary to take the sample from a relatively smooth and consequently pure part of the cathode, as otherwise it would not draw. The same with the mass of copper; you pick out a good solid part of the copper, which is not representative. In fact, the refiners have all found they could not take fair samples of cathodes for a chemical analysis. It is always customary to use wire-bar copper for the purpose of sampling, and the impurities are several times as great as shown by samples taken from the cathodes. I think if we examine all of the examples he has given of cases where copper is higher in conductivity, it will fit with that explanation. For example, the Elmore process is a direct electrolytic deposit. Incidentally, at the present time there are a number of experiments going on on the question of electrolytic wire which look quite promising.
About the oxygen content of copper, copper dissolves gases the same as other metals when it is molten. Any of you who have worked with silver know how it spits as it cools, every one knows how iron “pipes,” and copper does exactly the same thing. I am not sure it is oxygen it absorbs; it may just as well be CO or CO2; but there is no difficulty in getting rid of the oxygen in the present furnace. The only difficulty is than when the oxygen is poled out the copper “spews.” I have taken copper wire bars with practically no oxygen, which were in horrible shape physically, and they rolled perfectly as far as conductivity goes, and there was no trouble at all with the copper, so that if we melted copper in a neutral atmosphere it does not follow that we would be able to do without the cuprous oxide and get a perfect casting.